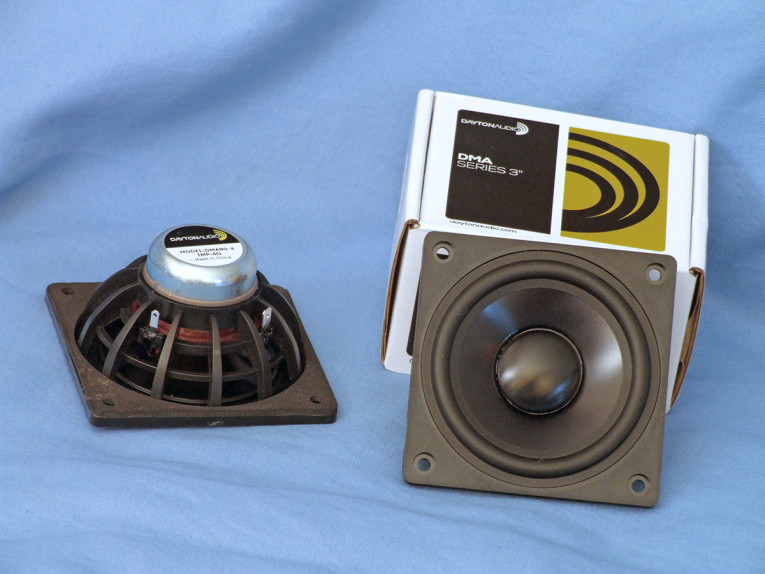
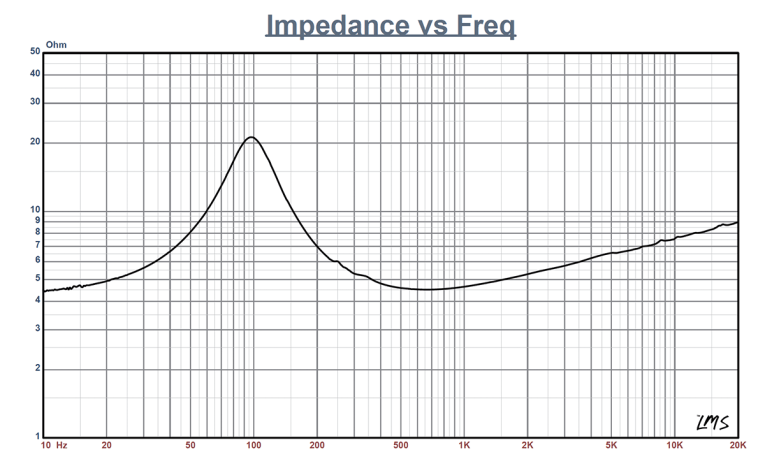
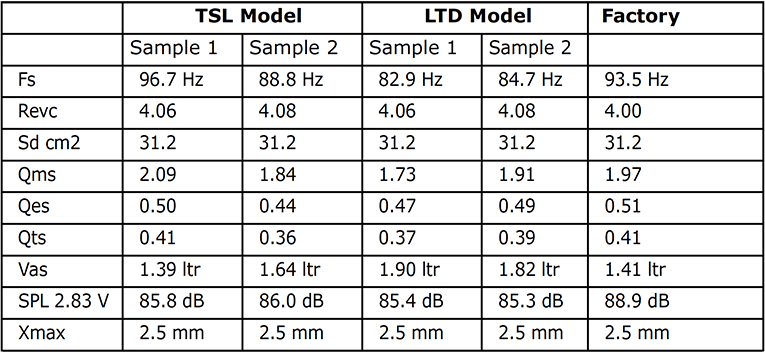
All five models have pretty much the same feature set starting with a proprietary 12-spoke injection-molded frame. This frame is very open to reduce reflections back into the cone and also has a very generous mounting flange making multiple driver arrays cosmetically attractive and compact. The DMA80 cone assembly consists of a black anodized aluminum cone, with a 26 mm diameter convex aluminum dust cap (directly coupled to the voice coil former), suspended with NBR and a 45 mm diameter treated cloth surround spider (damper) for compliance.
Motive force for the cone assembly is supplied by a neodymium slug-type motor with an additional bucking magnet to focus flux into the gap area. Driving the assembly is a 1” aluminum vented (five vents below the spider mounting shelf and eight smaller vents above it) former wound with two layers of round copper wire. Other features include a large copper cap shorting ring (Faraday Shield) that lowers distortion and extends the high-frequency SPL profile. Tinsel leads connect on one side of the cone to a pair of solderable terminals.
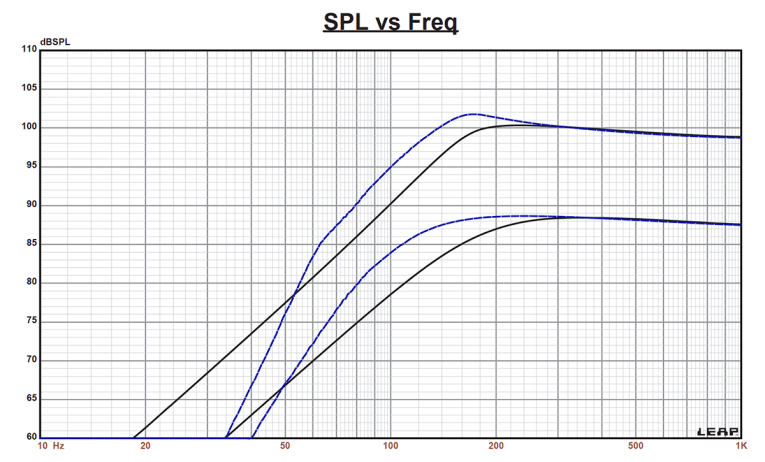
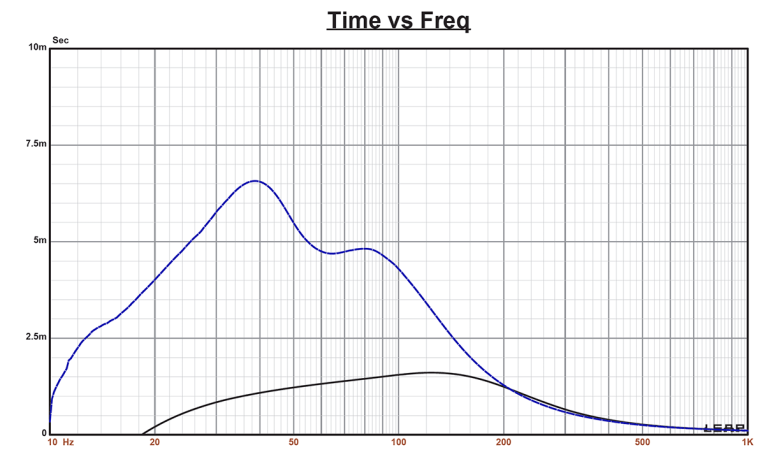
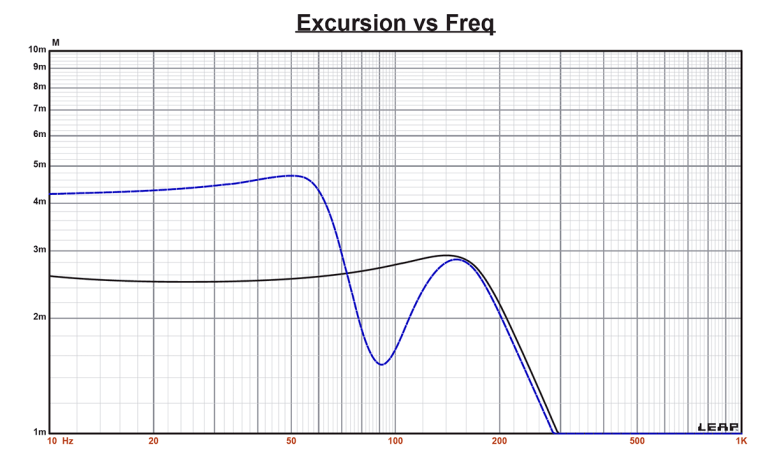
I commenced testing the Dayton Audio DMA80 full-range using the LinearX LMS analyzer and VIBox to create both voltage and admittance (current) curves with the driver clamped to a rigid test fixture in free-air at 0.3V, 1V, 3V, and 6V. It should also be noted that this multi-voltage parameter test procedure includes heating the voice coil between sweeps for progressively longer periods to simulate operating temperatures at that voltage level (raising the temperature to the first and second time constants).
Next, I post-processed the six 550-point stepped sine wave sweeps for each of the DMA80 samples and divided the voltage curves by the current curves (admittance) to produce the impedance curves, phase generated by the LMS calculation method. I imported the data, along with the accompanying voltage curves, to the LEAP 5 Enclosure Shop software.
I then selected the complete data set, the multiple voltage impedance curves for the LTD model, and the 1V impedance curve for the TSL model in the transducer derivation menu in LEAP 5 created the parameters for the computer box simulations. Figure 1 shows the 1V free-air impedance curve. Table 1 compares the LEAP 5 LTD and TSL data and factory parameters for both of the Dayton Audio DMA80 samples.
The LEAP TSL/LTD parameter calculation results for the DMA80 were reasonably close to the factory data. As always, I followed my usual protocol and set up computer enclosure simulations using the LEAP LTD parameters for Sample 1. This consisted of a 38 in3 Butterworth sealed box with 50% damping material (fiberglass), and a 61 in3 Quasi third-order Butterworth (QB3) vented alignment tuned to 89Hz with 15% damping material in the box.
Note that I use the simulated fiberglass (R19) selection in LEAP 5 primarily because it is accurate and a known commodity in terms of damping. However, due to its controversial carcinogenic nature, fiberglass is almost never used anymore — although I have seen it in the past few years in the pro market. Most damping material today is either foam or some form of Dacron batting. While LEAP does model polyester, the density is not as well established as fiberglass.
Figure 2 displays the results for the DMA80 in the sealed and vented box simulations at 2.83 V and at a voltage level sufficiently high enough to increase cone excursion to Xmax + 15% (2.88 mm for the DMA80). This produced a F3 frequency of 112 Hz (F6 = 129 Hz) for the 38 in3 sealed enclosure and –3 dB = 112 Hz (-6 dB =92 Hz) for the 61 in3 QB3 vented simulation.
Increasing the voltage input to the simulations until the maximum linear cone excursion was reached resulted in 100.5 dB at 15 V for the sealed enclosure simulation and 102 dB with the same 15 V input level for the vented enclosure. Figure 3 shows the 2.83 V group delay curves. Figure 4 shows the 15 V excursion curves. Again, as for any vented enclosure, a high-pass filter is highly desirable.
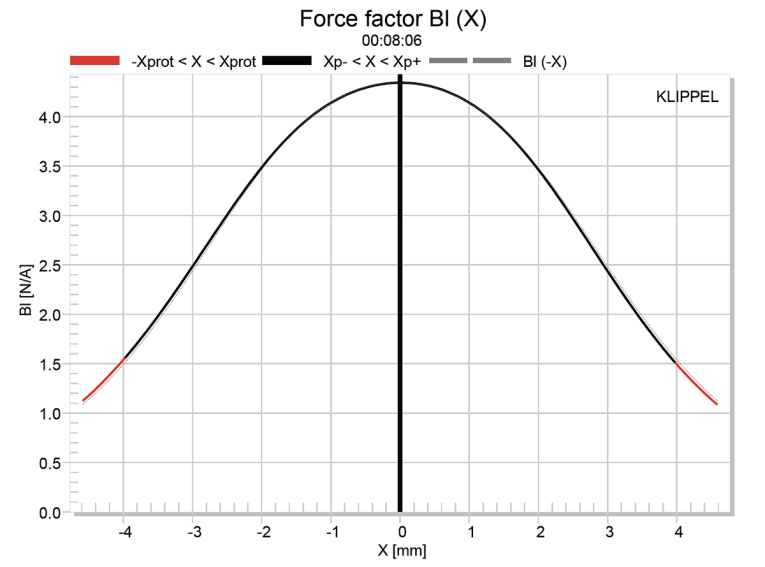
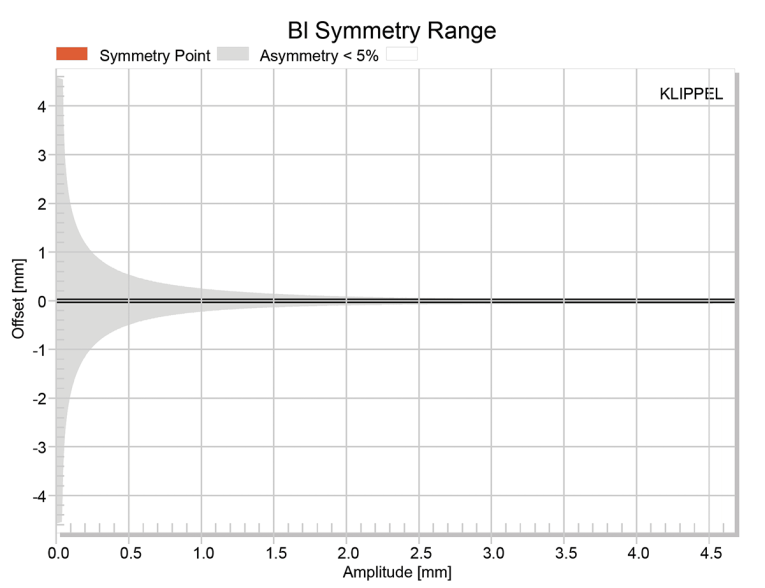
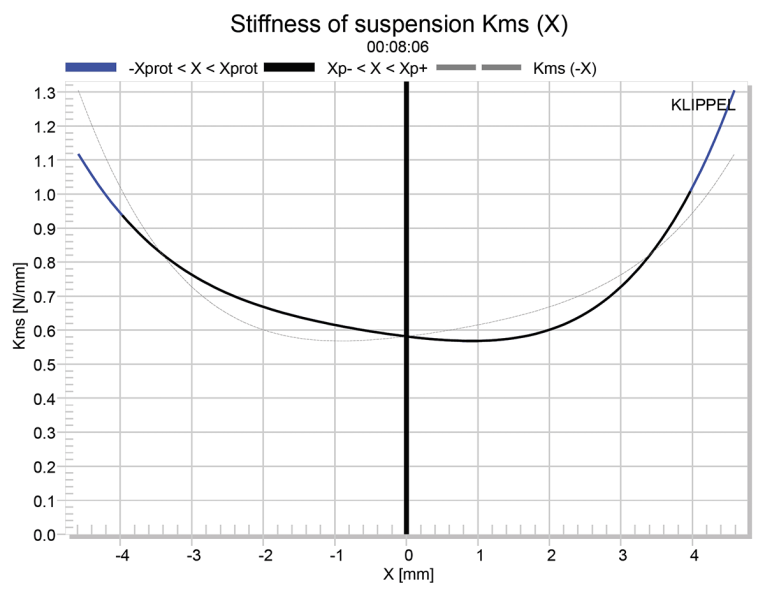
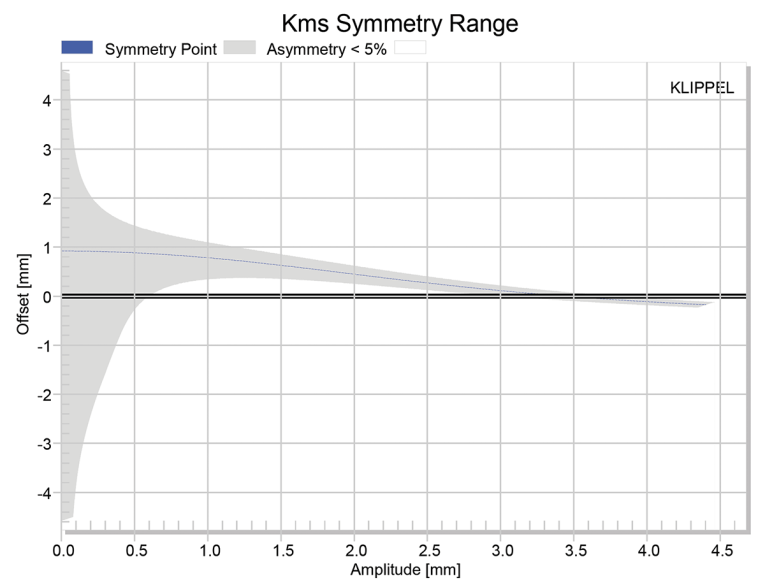
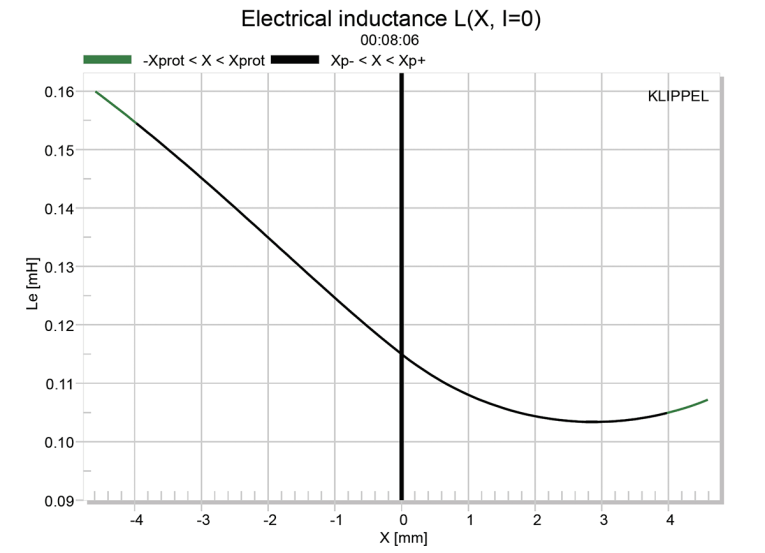
Klippel analysis for DMA80 produced the Bl(X) and Kms(X) shown in Figures 5-8. The Bl(X) curve for the DMA80 (see Figure 5) is typical for a short Xmax driver and is very symmetrical with little or no offset. Looking at the Bl symmetry plot (see Figure 6), this curve validates the Bl(X) curve and also shows virtually no offset. The stiffness of suspension Kms (X) curve (Figure 7) exhibits a degree of asymmetry, tilt, and offset. Looking at the Kms symmetry range curve (Figure 8), at a point of reasonably certainty at 1.5 mm the driver has a relatively minor 0.63 mm forward (coil out) movement, which decreases to 0.27 mm at the physical 2.5 mm Xmax point.
Figure 9 gives the DMA80’s inductance curve L(X). Inductance will typically increase in the rear direction from the zero rest position, which is what happens here. However, the DMA80 incorporates a copper shorting ring so the inductive swing is very small. Inductance variation for the DMA80 is only 0.14 mH to 0.10 mH from the coil-in and coil-out Xmax positions, which is good.
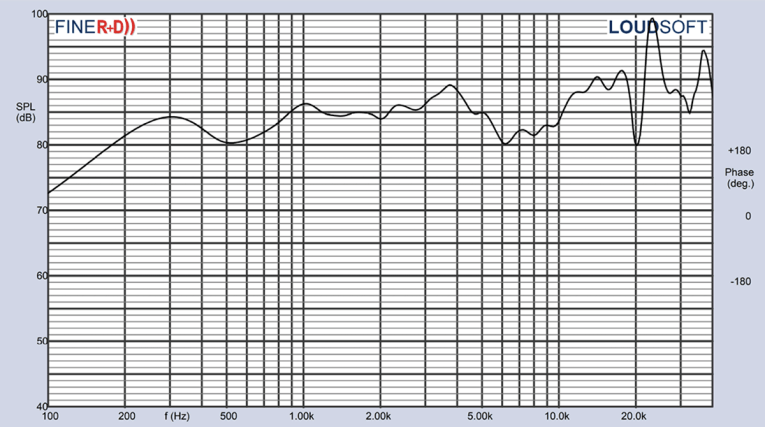
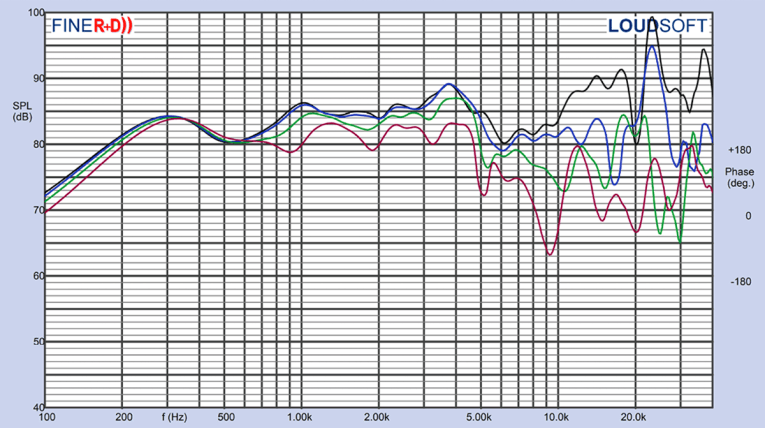
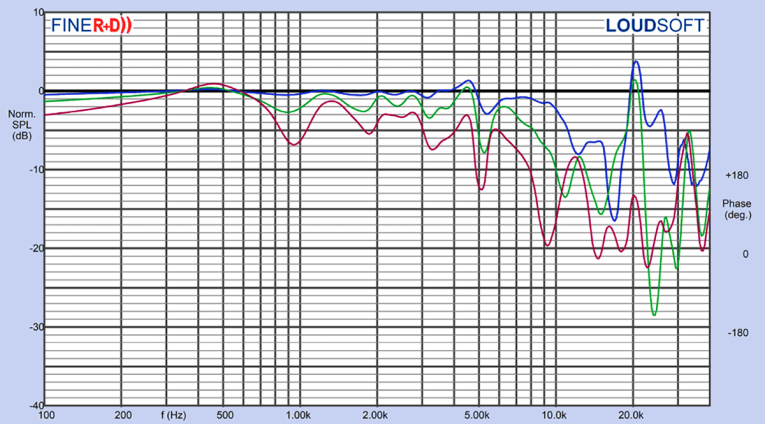
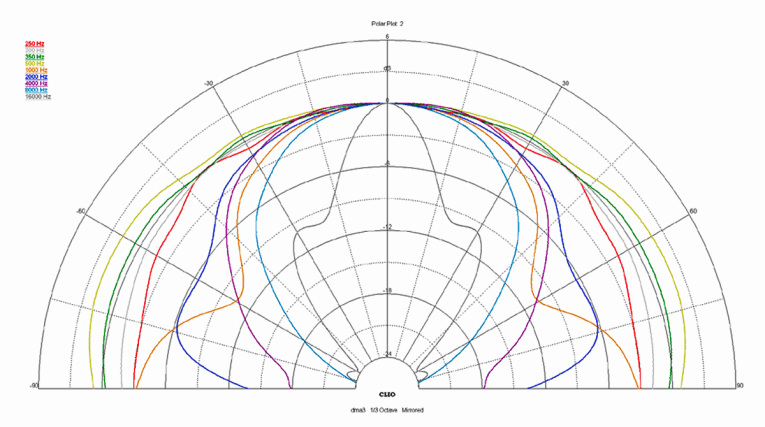
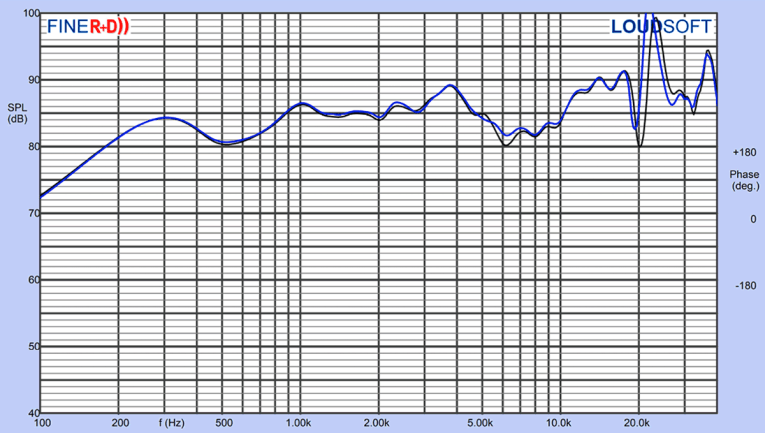
Next I mounted the DMA80 in an enclosure that had a 9” × 4” baffle and was filled with damping material (foam). Then, I measured the transducer on and off-axis from 300 Hz to 40 kHz frequency response at 2.83 V/1 m using the 192 kHz Loudsoft FINE R+D analyzer and the GRAS Sound & Vibration 46BE microphone. Figure 10 gives the DMA80’s on-axis response indicating a ±4.5dB SPL variation from 300 Hz to 13.5 kHz with sharp break up mode peaking modes at 23 kHz.
Figure 11 displays the on- and off-axis frequency response at 0°, 15°, 30°, and 45°. Figure 12 gives the off-axis curves normalized to the on-axis response. Figure 13 shows the Audiomatica CLIO 180° polar plot (measured in 10° increments). Figure 14 shows the two-sample SPL comparison, indicating the two samples are closely matched with 0.5 dB, except for a small area centered on 6 kHz.
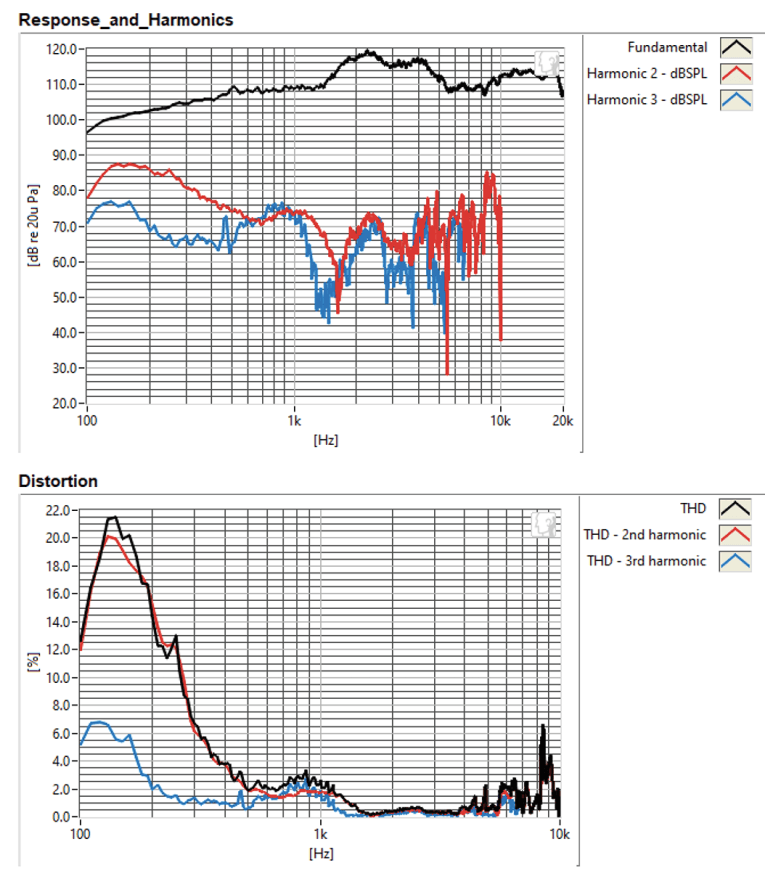
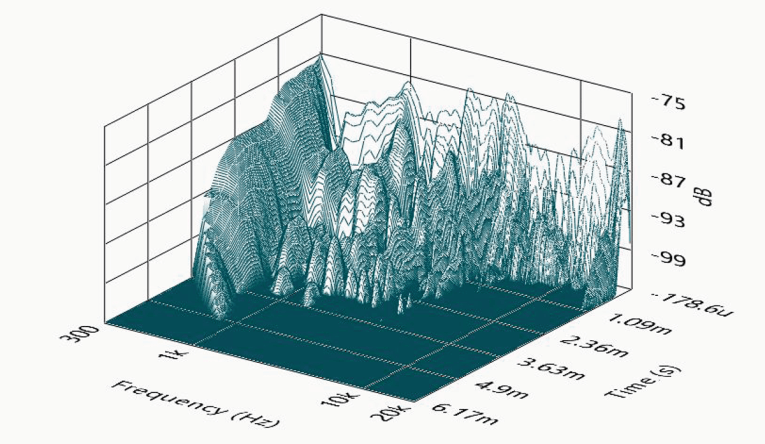
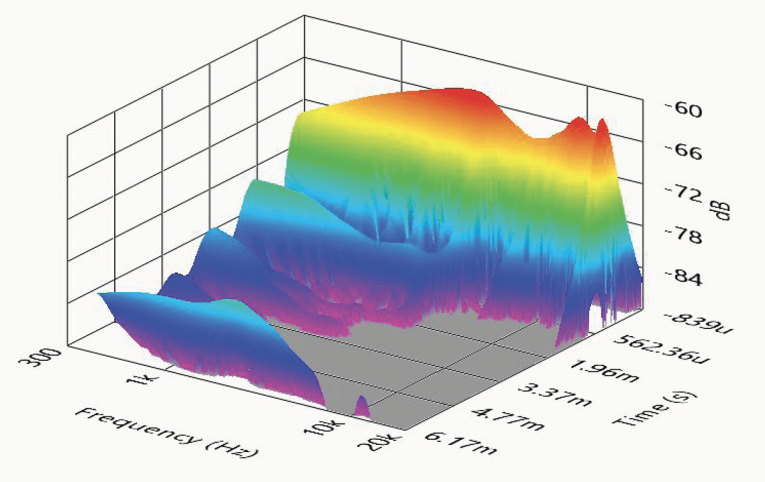
For the remaining series of tests, I employed the Listen AudioConnect analyzer operating with SoundCheck 17 software along with the Listen Inc. 1/4” SCM microphone (courtesy of Listen, Inc.) to measure distortion and generate time-frequency plots. For the distortion measurement, I mounted the DMA80 rigidly in free-air and used a pink noise stimulus to set the SPL to 94 dB at 1 m (7.55V). Then, I measured the distortion with the microphone placed 10 cm from the dust cap. This produced the distortion curves shown in Figure 15.
I then used SoundCheck 17 to get a 2.83 V/1 m impulse response and imported the data into Listen’s SoundMap Time/Frequency software. Figure 16 shows the resulting CSD waterfall plot. Figure 17 shows the Wigner-Ville plot (for its better low-frequency performance). The performance of the DMA80 is quite good for this small a driver, and should be quite effective in array applications. For more information, visit www.daytonaudio.com. VC
This article was originally published in Voice Coil, November 2019.