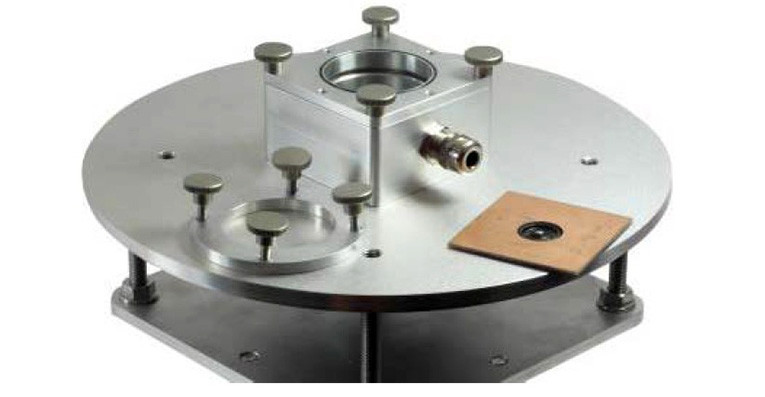
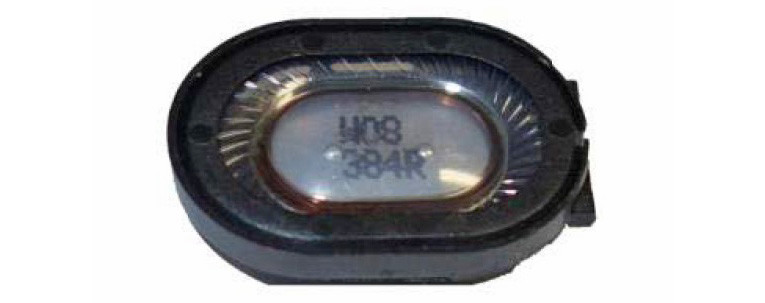
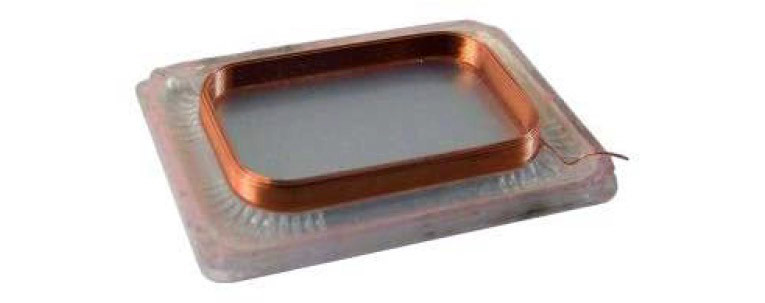
To consider damping and visco-elasticity in the material, a dynamic measurement is required that uses an AC stimulus comprising signal components in the audio band. The Micro Suspension Part Measurement (MSPM) technique uses dynamic identification and provides the parameters of a small diaphragm/suspension without utilizing an assembled transducer. This article will discuss the measurement method using the MSPM bench shown in Photo 1. We will also compare it to the traditional methods using a microspeaker as the target transducer (see Photo 2).
Relevant Suspension Part Parameters
Reducing the descriptors of suspension behavior to fewer, more informative parameters is elemental for a more robust specification of these parts. In this method, the parameters are divided into linear and nonlinear parameters. The linear parameters describe the mechanical resonator at small amplitudes as a damped spring-mass system. The mass “m” and stiffness “K” define the resonance frequency “fres.” The Q-factor depends on the mechanical resistance “R.” The microspeaker’s linear characteristics, divided into the measured parameters, can be seen in Table 1. In this case, the suspension was measured in an assembled transducer. Therefore, the results include the voice coil’s mass and possible losses caused by the air in the gap.
This technique can be also applied to the separated diaphragm as shown in Photo 3. Due to the geometry and the suspension’s specific material, the stiffness “K” is usually not constant. Instead, it depends on the instantaneous displacement “x,” time “t,” frequency “f,” and the ambient conditions (temperature and humidity). The dependency of K(x) on displacement “x” causes one of the dominant nonlinearities inherent in loudspeakers, generating distortion for any excitation signal below resonance. A typical curve K(x) is shown in Figure 1.
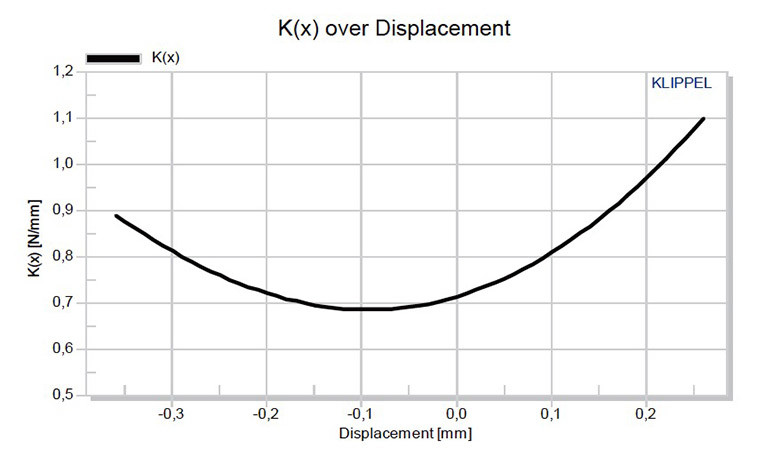
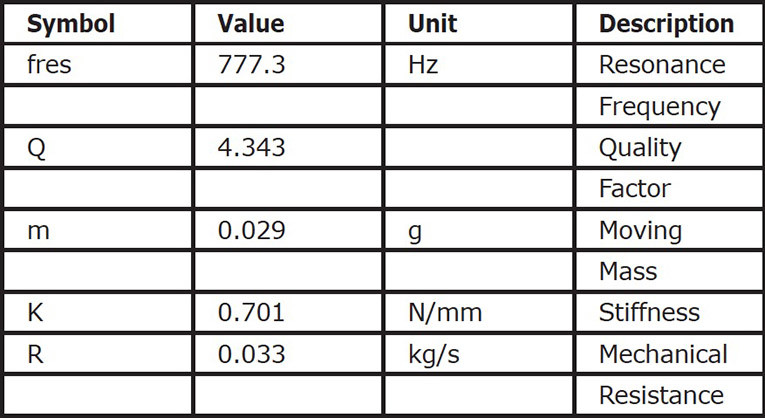
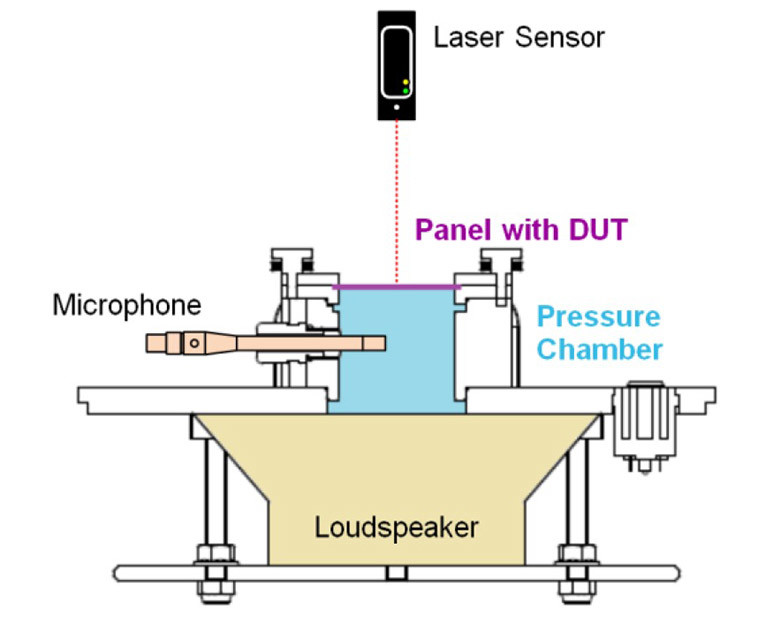
Dynamic Measurement Technique
Traditional methods calculate suspension by measuring the resulting restoring force caused by a displacement x, using a mechanical device. This method could be found lacking for several reasons.
When testing soft parts (e.g. diaphragms), the measurement devices will typically inject force at a point in the middle of the membrane. This will, especially for thin diaphragms (e.g., the ones used in micro-speakers and headphones), deform the diaphragm, altering the measurement results. A second reason is that the viscoelastic creep will create a frequency dependent stiffness. In a static measurement, the suspension will be measured at a very low frequency. Therefore, a different stiffness is measured, much lower than the final value seen in the end application.
A dynamic measurement is required for an accurate parameter measurement when the suspension part is glued or clamped into a panel and clamped onto a sealed pressure chamber. The diaphragm is deflected by the sound pressure generated by a sensitive midrange transducer as used in horn-loaded PA equipment. The identification of the parameters requires the measurement of some state variables such as force, displacement, or pressure in the system. A direct measurement of the total driving force “F” is not possible. However, the displacement and the sound pressure in the box can easily be measured using a laser triangulation sensor and a microphone mounted inside the box (see Figure 2).
The linear parameters are determined using the added mass perturbation method. Applying a sweep signal, the suspension is passively excited, enabling the measurement of the resonant frequency “fres” and Q-factor. A known mass is then added and the parameters are identified by measuring the resonance shift (see Figure 3).
Detailed information about the suspension’s properties highlights the stiffness’s dependence on displacement K(x). The stiffness curve is calculated using the nonlinear distortion found in the displacement signal, determined by the laser. By identifying the nonlinear model of the displacement varying stiffness, the driving force is modeled, which is proportional to sound pressure inside the pressure chamber. The difference between measured and predicted sound pressure is used as an error signal for nonlinear system identification (see Figure 4). Least-Mean-Square algorithm (LMS) minimizes the error and calculates the nonlinear curve shape by exploiting the nonlinear distortion found in the measured displacement (see Figure 5).
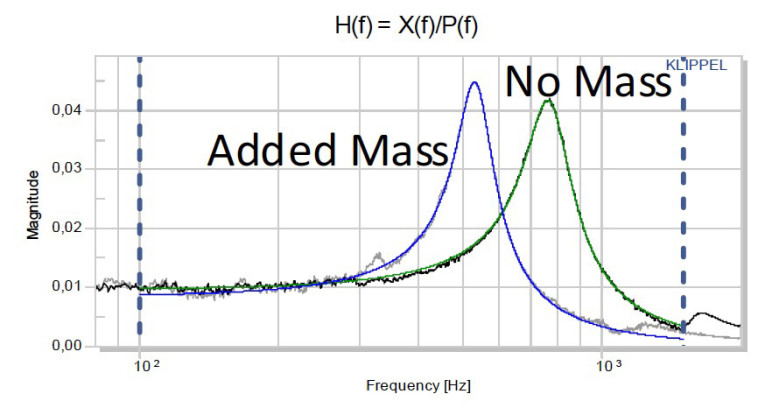
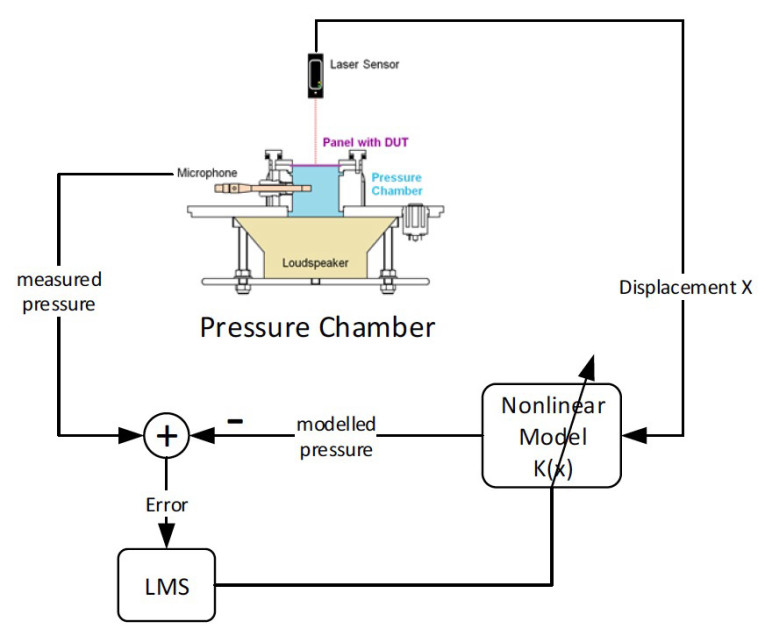
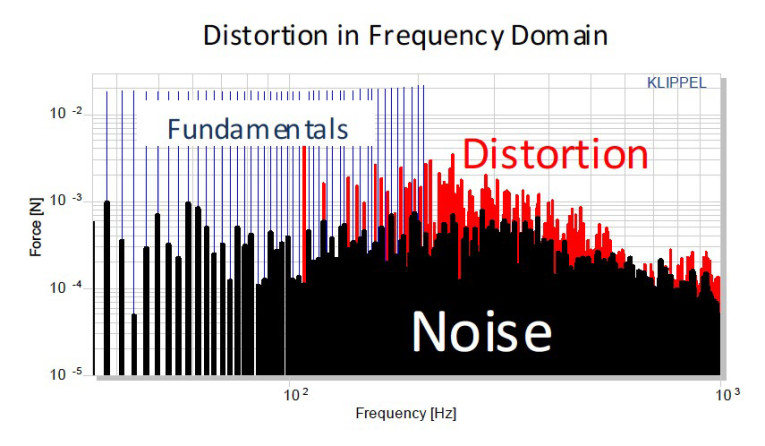
Comparison with Speaker Application
This measurement technique’s main goal is to identify the suspension part parameters in the final driver application. The results of the MSPM technique show a good accordance with the parameters measured in the final application (see Figure 6).
The results generated by MSPM are comparable with the results generated by the Large Signal Identification (LSI) according to Standard IEC 62458 based current monitoring at the loudspeaker terminals. Figure 6 shows minor differences in the results are caused by different excitation conditions, temperature, humidity, and viscoelasticity.
The electrical excitation of the suspension part generates a force at the voice coil position, whereas the pneumatical excitation of the MSPM generates forces distributed over the membrane area (see Figure 7). The influence of the measurement technique is negligible for transducers with rather stiff center parts and softer suspension regions on the diaphragm.
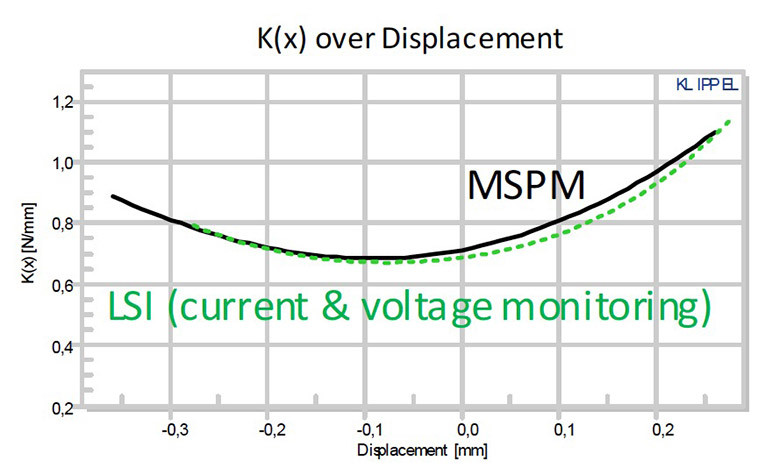
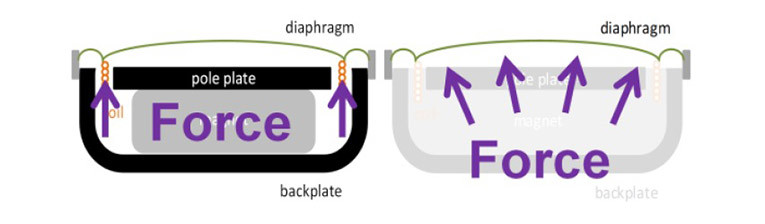
Summary
Suspension part measurements for microspeakers, headphones, tweeters, and microphones provide several benefits including:
• Enable the individual measurement of stiffness and damping for material and diaphragm producers.
• Enable faster development cycles by measurement of suspension parameters without the need of assembling the speaker. Suspension part measurements overcome the problems of traditional static measurements (viscoelastic creep).
• Complies with industry standard Large Signal Identification (LSI) method according to the IEC 62458 standard used for complete speakers
• Measure suspension parameters under real clamping conditions.
• Help identify asymmetrical suspension, which is the major source of substantial DC generation.
www.klippel.de
This article was originally published in Voice Coil, January 2016.