
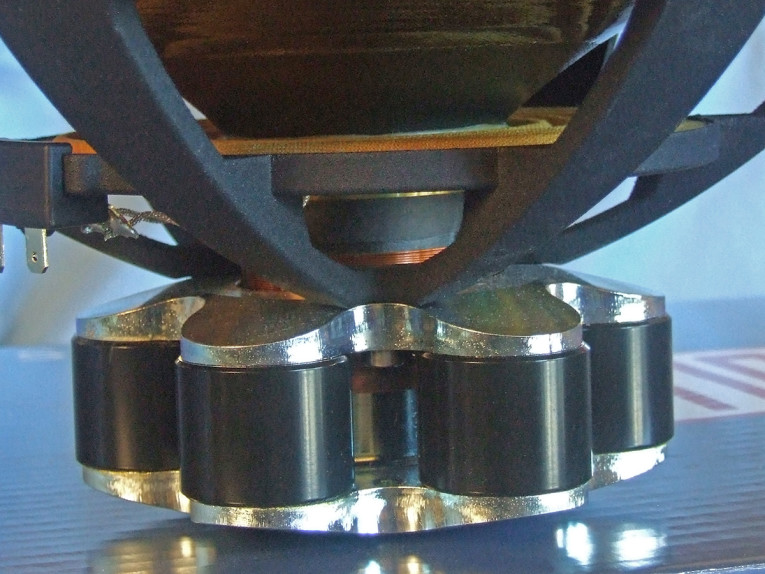
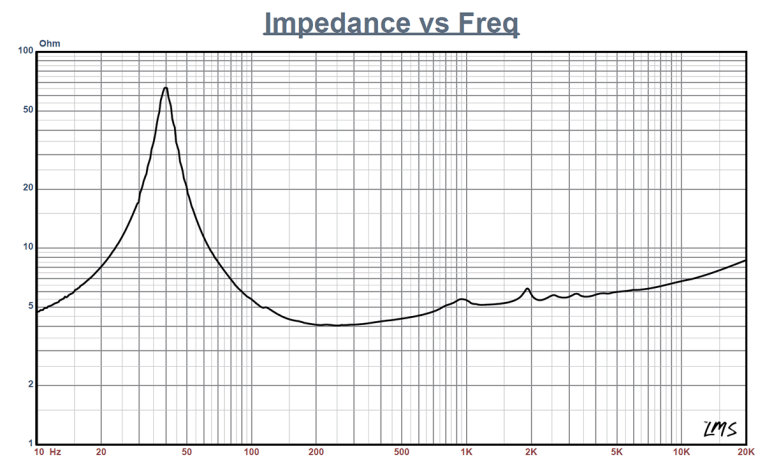
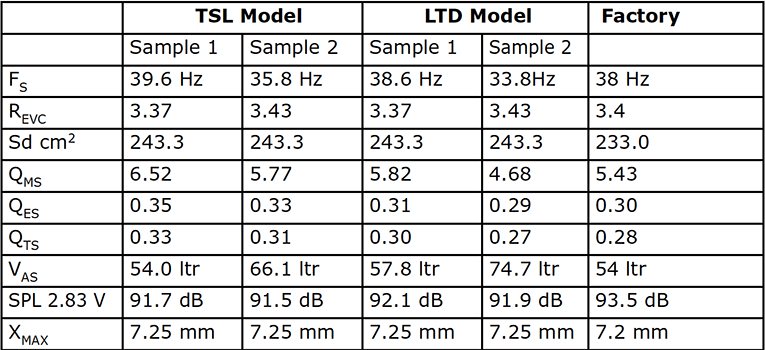
And, the first oval voice coil Scan-Speak Ellipticor drivers characterized in Test Bench were the 18WE/4542T00 and the D3404/552000 (Voice Coil, April 2018). Evidently, the idea of using elliptical-shaped voice coils to control cone and dome modes came from an engineering brain storming session that involved Birger Jorgensen (sadly, Jorgensen passed away nearly two years ago). One of the goals for a new driver concept at Scan-Speak was to minimize cone modes, so it was kind of a joke to suggest an elliptical voice coil, but it was engineering humor that had some solid science behind it. By driving the diaphragm asymmetrically using the oval voice coil concept, what you get is an “infinite” number of Eigen frequencies, but with less contribution for each frequency and overall lower distortion.
Driving the diaphragm symmetrically you get a finite number of Eigen frequencies with a higher contribution combining at certain frequencies producing the “dreaded” coloration modes. I should also note that the concept of using asymmetry to suppress diaphragm modes, not with an asymmetrical voice coil, but by using an asymmetrical diaphragm with a round coil was patented by my friend and consulting associate and extremely clever transducer engineer Enrique Stiles, in US Patent Number 7177440.
This month, Scan-Speak sent me the latest iteration of the Ellipticor product line, the 8” 21WE/4542T00 midbass woofer, which is the big brother to the 18WE/4542T00. As with the 18WE, the 21WE/4542T00 is a seriously feature rich transducer (see Photo 1). Starting with the frame, like most all of Scan-Speak’s midwoofer/midrange frames, the 21WE/4542T00’s frame is a really nicely configured six-spoke (three double spokes) cast-aluminum frame with narrow 19-10 mm wide spokes to minimize reflections back into the cone. The area below the suspended spider mounting shelf is almost completely open (see Photo 2), resulting in effective cooling of the motor and voice coil.
For the cone assembly, Scan-Speak chose a rather stiff curvilinear profile black-coated paper cone with an elliptical 45 mm × 35 mm convex paper dust cap. Compliance is provided by a nitrile rubber (NBR) surround, nicely designed with a fairly shallow discontinuity where it attaches to the cone edge, plus a surface coating for enhanced damping in that critical region. Remaining compliance comes from a 4.4” diameter flat cloth spider.
The motor design for the Ellipticor 21WE/4542T00 is nearly identical to the 18WE (with nearly the same Bl) and is also an AirCirc multi-magnet design utilizing six 22mm high and 25mm diameter neodymium slugs with a black emissive coating. This is also one of Scan-Speak’s patented SD motor systems and incorporates copper shorting rings (Faraday shields) also visible in Photo 2. FEA designed, the neodymium magnet motor uses a 35mm × 45mm elliptical two-layer voice coil wound with round copper wire on a titanium former (also identical to the 18WE). Motor parts include polished front and back plates for the neodymium magnets that incorporates a 12mm diameter rear vent for additional cooling.
As with all the transducers in Ellipticor lineup, the 21WE/4542T00 has a magnetically attached aluminum trim ring that can obviously be custom anodized in a variety of colors besides black. Last, the voice coil is terminated to a pair of gold-plated terminals. In terms of physical appearance, this driver is cosmetically quite attractive.
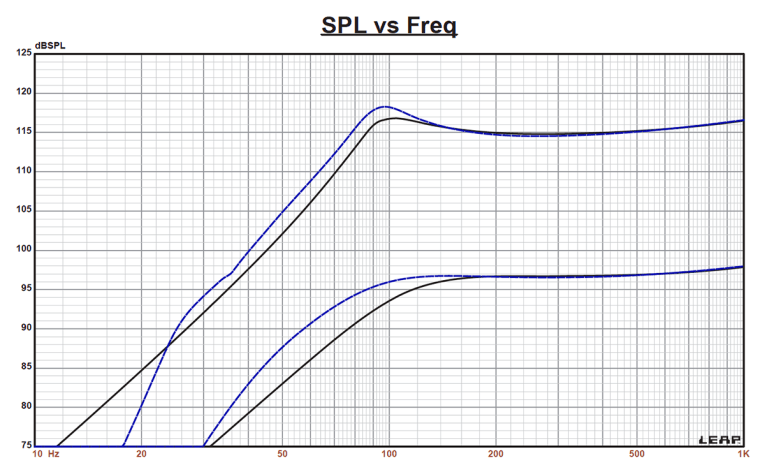


I began analysis of the 21WE/4542T00 using the LinearX LMS analyzer and VIBox to create both voltage and admittance (current) curves with the driver clamped to a rigid test fixture in free-air at 0.3V, 1V, 3V, 6V, 10V, and 15V. As with almost all 8” diameter woofers, the driver stayed sufficiently linear out to 15V for LEAP to get a reasonable curve fit. As has become the protocol for Test Bench testing, I no longer use a single added mass measurement to determine Vas. Instead, I use the actual measured cone assembly mass (Mmd) supplied by the driver manufacturer, which was 22.96 grams for the 21WE/4542T00.
Next, I post-processed the 12 550-point stepped sine wave sweeps for each sample and divided the voltage curves by the current curves (admittance) to derive impedance curves, phase added by the LMS calculation method. Then, I imported the data, along with the accompanying voltage curves, to the LEAP 5 Enclosure Shop software.
Since most Thiele-Small (T-S) data provided by the majority of OEM manufacturers is generated using either the standard model or the LEAP 4 TSL model, I additionally created a LEAP 4 TSL parameter set using the 1 V free-air curves. I selected the complete data set, the multiple voltage impedance curves for the LTD model, and the 1 V impedance curve for the TSL model in the transducer derivation menu in LEAP 5 and created the parameters for the computer box simulations. Figure 1 shows the 1 V free-air impedance curve. Table 1 compares the LEAP 5 LTD, TSL, and factory published parameters for both samples.
LEAP parameter calculation results for the 21WE/4542T00 were sufficiently close to the published factory data, with the only variation being sensitivity. The number that I publish is a calculated midband SPL that results from T-S parameter routine, whereas Scan-Speak is using a 2.83 V/1 m response measurement average.
As usual, I followed my normal protocol and set up computer enclosure simulations using the LEAP LTD parameters for Sample 1. I programmed two computer box simulations into LEAP 5, one a Qtc = 0.7 Butterworth sealed box with a 0.4 ft3 volume with 50% fiberglass damping material; and a vented QB3 enclosure with a 0.59 ft3 volume tuned to 50 Hz with 15% fiberglass fill material.
Figure 2 displays the results for the 21WE/4542T00 in the vented and sealed boxes at 2.83 V and at a voltage level sufficiently high enough to increase cone excursion to Xmax + 15% (8.3 mm). This produced a F3 frequency of 101 Hz (F6 = 80 Hz) with a Qtc = 0.68 for the sealed enclosure and –3 dB = 74.5 Hz (F6 = 60 Hz) for the vented box. Increasing the voltage input to the simulations until the maximum linear cone excursion was reached resulted in 117 dB at 39.5 V for the sealed enclosure simulation and 119 dB for the same 39.5 V input level for the vented enclosure.
Figure 3 shows the 2.83 V group delay curves. Figure 4 shows the 39.5 V excursion curves. I limited the voltage on the vented simulation because the driver was started to over excurse below 35 Hz, and as with most all vented systems, should be bandlimited with a high-pass filter.

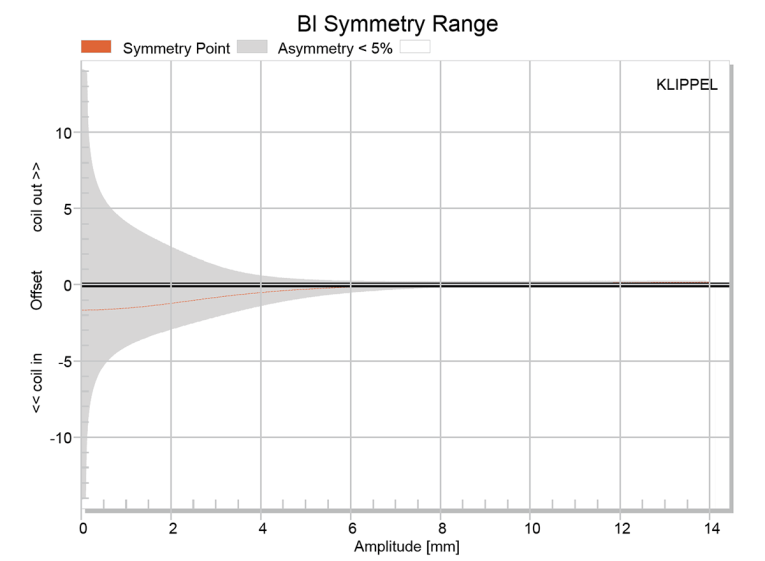
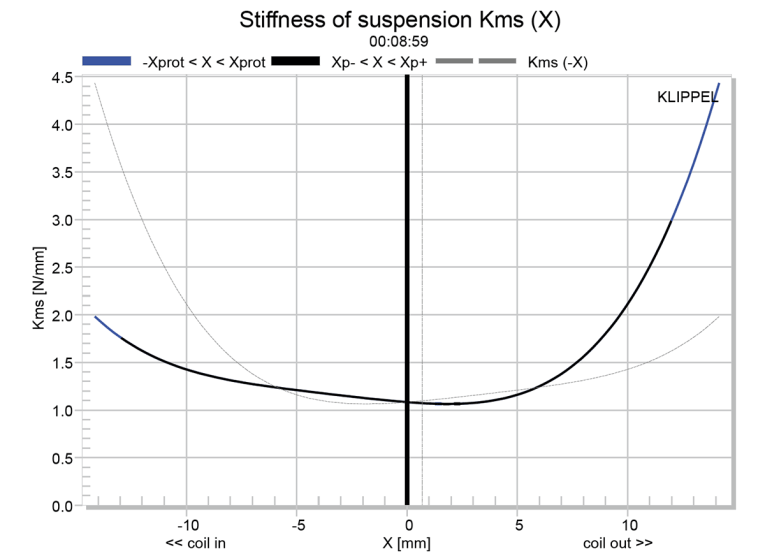
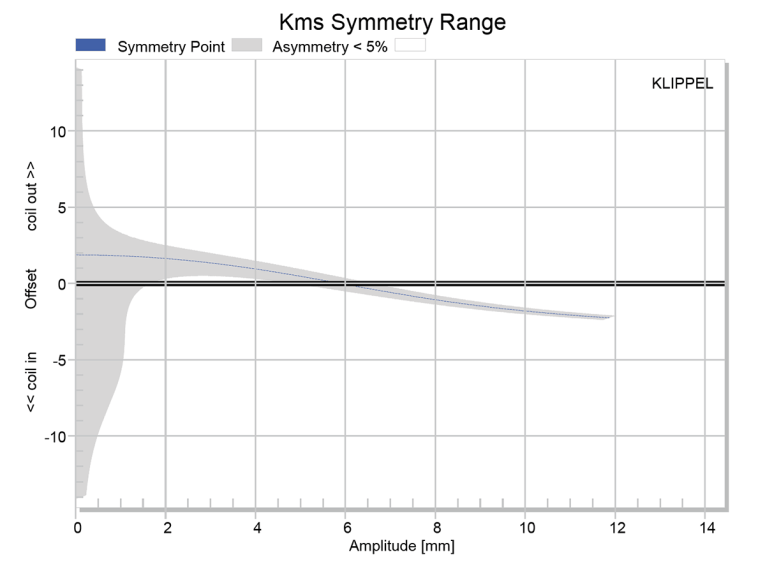
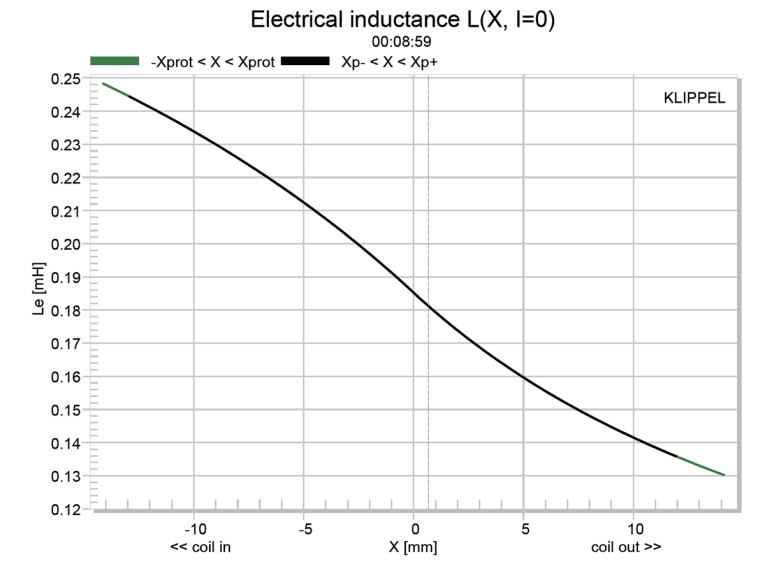
Klippel analysis for the 21WE/4542T00 produced the Bl(X), Kms(X), and Bl and Kms symmetry range plots given in Figures 5-8. (Our analyzer is provided courtesy of Klippel GmbH and the analysis is performed by Pat Turnmire, owner of Redrock Acoustics and author of the SpeaD and RevSpeaD software.) The Bl(X) curve for the 21WE/4542T00 (see Figure 5) is mostly symmetrical with a small amount of tilt and coil-in offset, however this is fairly broad Bl curve for an 8” woofer.
Looking at the Bl symmetry plot (see Figure 6), from an area of reasonable certainty (the grey overlay) at 4 mm, the coil-in offset is only 0.51 mm gradually decreasing coil-in offset to a meaningless 0.14 mm at the drivers Xmax +15% position (8.3 mm), which is no offset for all practical purposes. Figure 7 and Figure 8 show the Kms(X) and Kms symmetry range curves for the21WE/4542T00. The Kms(X) curve is definitely not as symmetrical in both directions as the Bl curve, and changes from coil-out to coil-in at about 6mm and is about 0.67mm at the physical Xmax position, which is not critical.
Displacement limiting numbers calculated by the Klippel analyzer for the 21WE/4542T00 were XBl at 82% Bl = 7.1mm and for XC at 75% Cms minimum was 7.4mm, which means that for this Ellipticor midwoofer, the Bl is the most limiting factor for prescribed distortion level of 10%. However, both numbers are at or near the physical Xmax, so really quite good. If we use a less conservative 20% distortion criteria of Bl falling to 70% of its maximum value and compliance falling to 50% of its maximum value, the numbers would be XBl = 8.6 mm and XC = 10.1, both numbers being greater than physical Xmax + 15% (8.3mm) of the driver.
Figure 9 gives the inductance curves Le(X) for the 21WE/4542T00. Inductance will typically increase in the rear direction from the zero rest position as the voice coil covers more pole area, which is what you see here. However, because of the effective use of copper shorting rings (Faraday shields), the inductive swing from Xmax in to Xmax out is a very small 0.072 mH, which is very good performance.
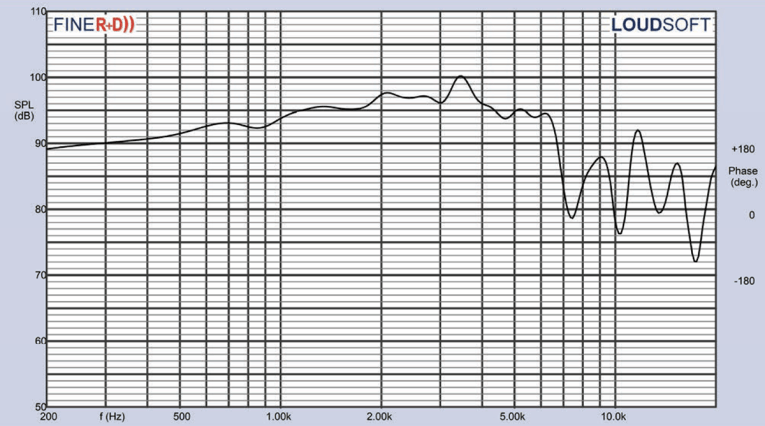
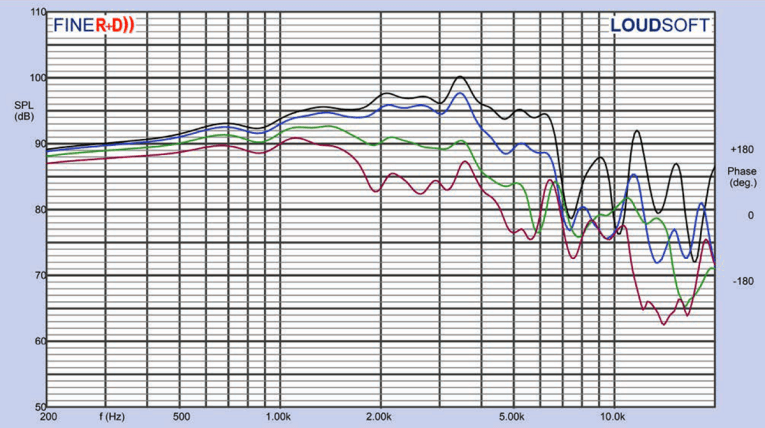
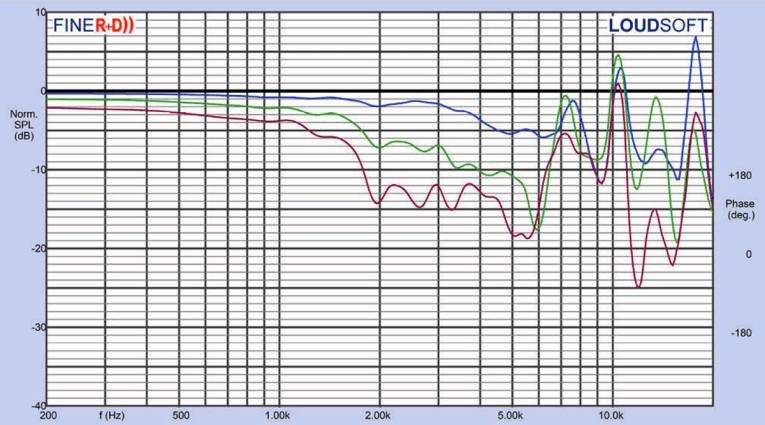
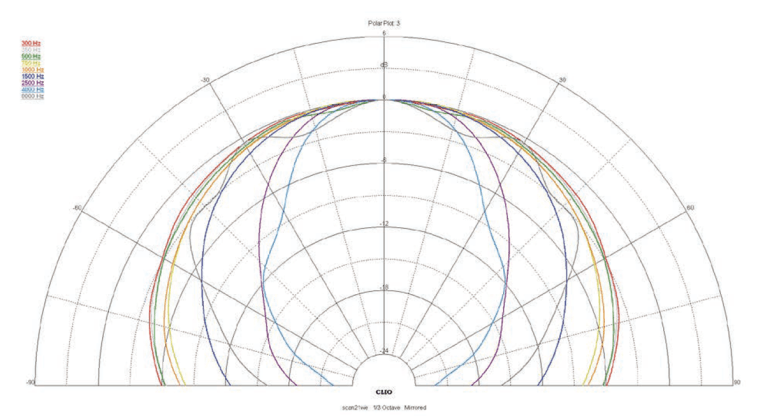

With the Klippel testing completed, I mounted the 21WE/4542T00 in an enclosure that had a 15” × 8” baffle and was filled with damping material (foam). Then, I measured the device under test (DUT) on- and off-axis from 300Hz to 40kHz frequency response at 2.00 V/0.5m normalized to 2.83 V/1m, using the LoudSoft FINE R+D FFT analyzer.
Figure 10 gives the 21WE/4542T00’s on-axis response indicating a smoothly rising response to about 3kHz with a small 4dB peak at 3.5 kHz where it begins its low-pass roll-off. Figure 11 displays the on- and off-axis frequency response at 0°, 15°, 30°, and 45°. The -3dB frequency at 30° off-axis relative to the on-axis SPL is about 1.5kHz, suggesting a crossover between 1.0 to 1.5kHz should be appropriate. The normalized version of Figure 11 is shown in Figure 12. Figure 13 shows the CLIO polar plot (done in 10° increments). And, Figure 14 gives the two-sample SPL comparisons for the 21WE/4542T00, showing a close match (less than 0.8 dB variation) throughout the operating range.
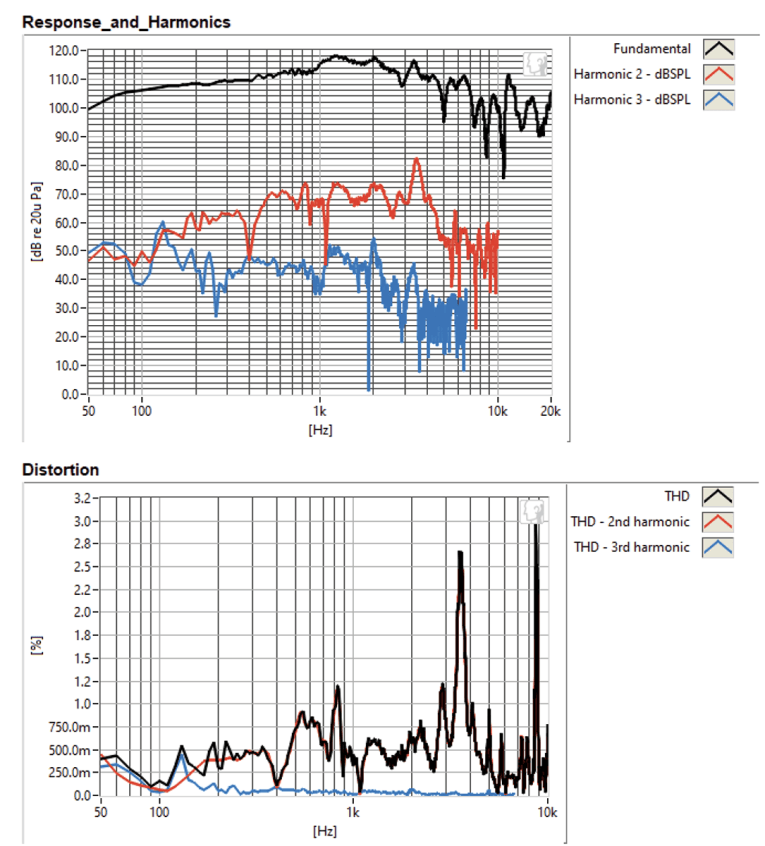

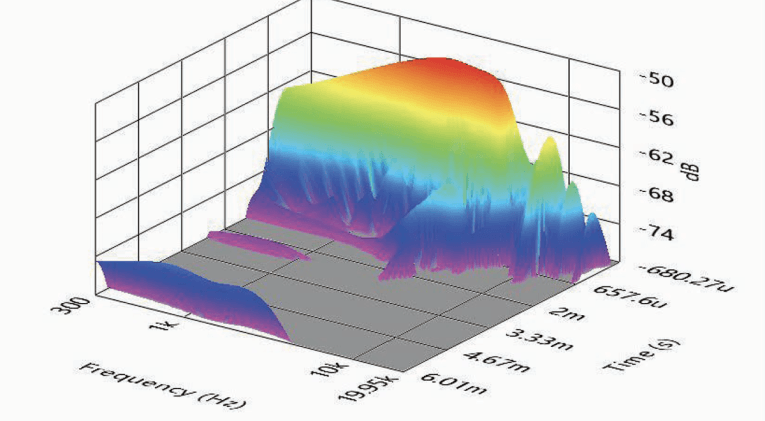
For the remaining series of tests, I set up the Listen, Inc. SoundCheck 18 software, AudioConnext analyzer and 1/4” SCM microphone (courtesy of Listen, Inc.) to measure distortion and generate time-frequency plots. For the distortion measurement, I mounted the 21WE/4542T00 rigidly in free-air, and set the SPL to 94dB at 1m (3.01V) using the pink noise generator and SLM built into the SoundCheck 18 software. Then, I measured the distortion with the Listen microphone placed 10 cm from the dust cap. This produced the distortion curves shown in Figure 15. As with the Scan-Speak 18WE, the third-order harmonic distortion was extremely low.
For the last test, I used SoundCheck 18 to obtain a 2.83 V/1m impulse response and import that data into Listen’s SoundMap Time/Frequency software. Figure 16 shows the resulting cumulative spectral decay (CSD) waterfall plot. Figure 17 shows the Wigner-Ville plot (for its better low-frequency performance).
The test results for this unique oval-shaped voice coil transducer indicate very good performance. While I have, as yet, no experience designing the 21WE/4542T00 into a system, I have managed to design a product using both the Ellipticor 18WE and the D3404, and in my opinion, the results are extremely musical sounding. For more information, visit www.scan-speak.dk. VC
This article was originally published in Voice Coil, October 2020.