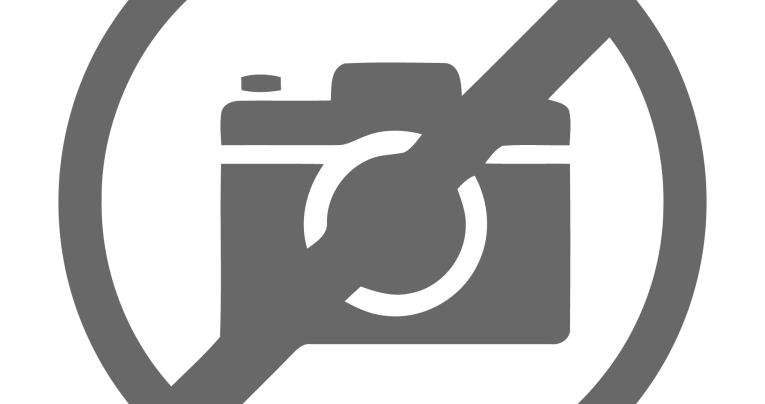
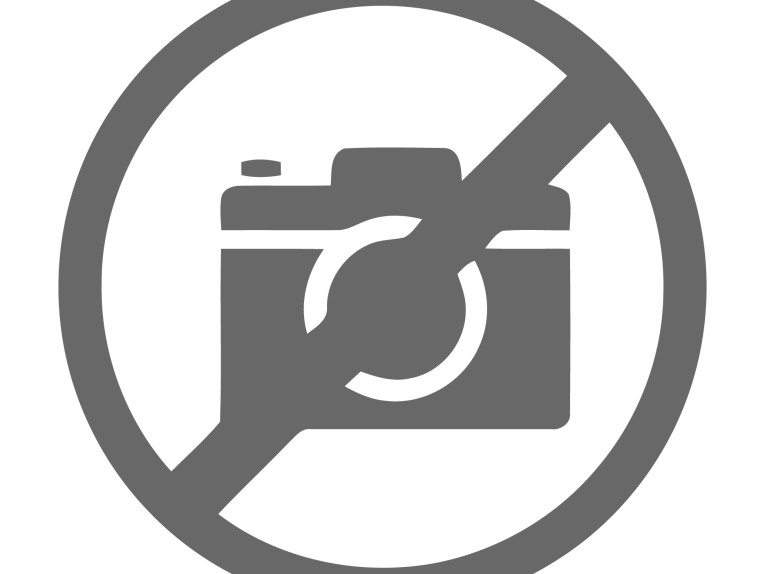

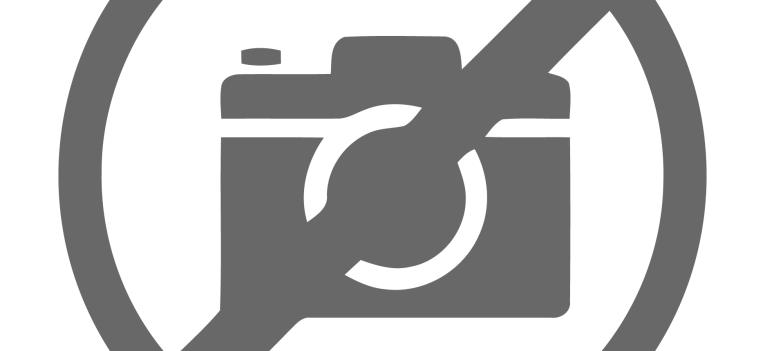
Starting with the BWX-6501 (originally known as Model 82109), this 6.5" woofer has a substantial feature set that begins with a proprietary 12-spoke cast-aluminum frame, comprised of a set of four groups of three closed narrow (about 4mm) tapered spokes, and includes 16 8mm × 4mm vents below the spider (damper) mounting shelf. Additional cooling for this driver is provided by eight 5mm diameter peripheral vents in the motor return cup, as seen in the cutaway drawing shown in Figure 1.
The cone assembly consists of a curvilinear profile Abaca fiber (paper) cone and a 37mm (1.46") diameter black aluminum phase plug surrounded by a soft trim piece attached to the cone (Photo 1). Compliance is provided by a NBR surround that has a nice shallow transition to the cone attachment, with the remaining compliance coming from a 3.5” diameter flat cotton spider (damper).
The XBL2 motor design is licensed from inventor Dan Wiggins for both of the new Bold North Audio woofers. Like the Mmag patented dual gap dual magnet motor technology developed by Enrique Stiles and Patrick Turnmire, the XBL2 motor features a short voice coil traveling between two gaps. While Mmag uses two magnets and two plates, XBL2 creates two gaps generally by using a thick front plate with a CNC’d groove in the middle of the plate to form the dual-gap structure. However, the BWX-6501 forms the gap with two milled front plates. Whichever dual-gap technology is used, Mmag or XBL, results in a typically shorter voice coil that travels into the second gap as it is traveling out of the first gap—the upshot being a higher Xmax (10mm for the BWX-6501) than most conventional motors can provide.
The BWX-6501’s two milled front plates are fitted with dual inside and outside copper shorting rings (Faraday shields). Driving the cone assembly is a 38.1mm (1.5”) diameter voice coil wound with round copper-clad aluminum wire (CCAW) on a non-conducting Nomex former. Last, the driver’s IEC268-5 power handing is rated at 85W and the voice coil is terminated to standard solderable terminals.
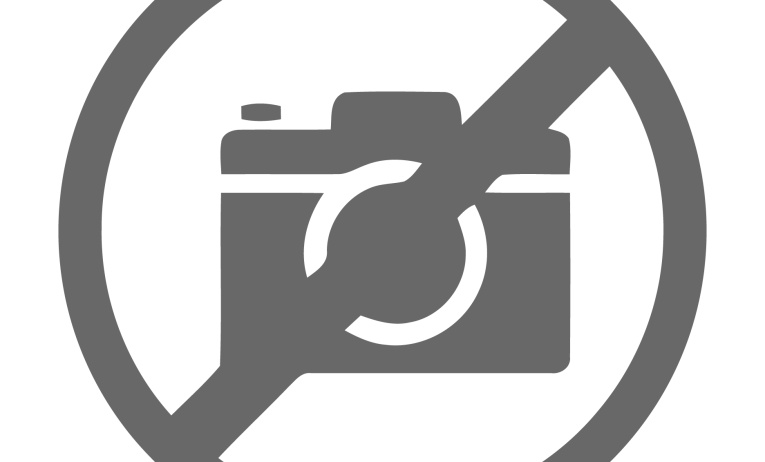
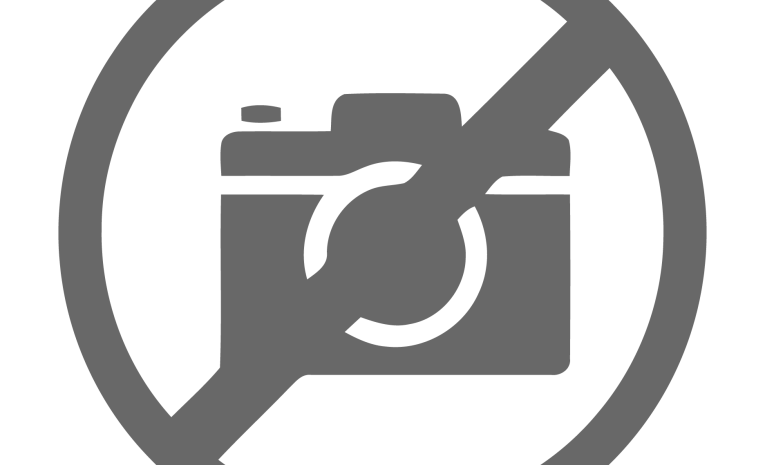
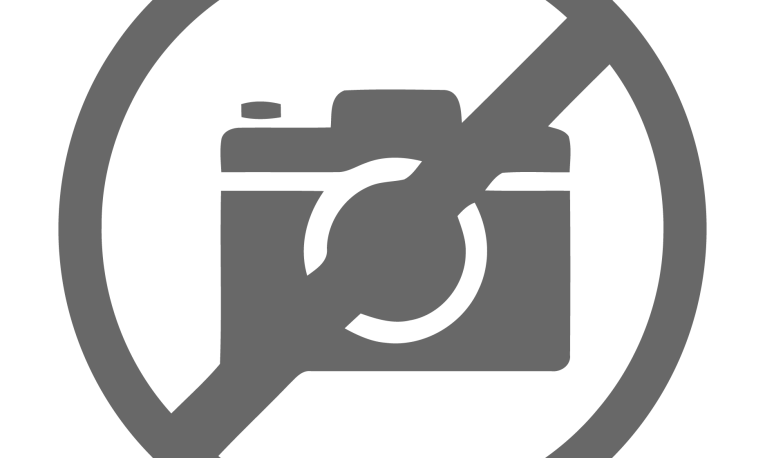
I began testing the Bold North BWX-6501 woofer using the LinearX LMS analyzer and VIBox to create both voltage and admittance (current) curves with the driver clamped to a rigid test fixture in free-air at 0.3, 1, 3, 6, 10, 15, and 20V. The BWX-6501 pretty much set a Test Bench record for the highest useable voltage sweep (20V) for a 6.5” driver ever since Test Bench began using the LinearX VIBox for multiple voltage impedance testing in October 2003. While I did not end up using the 20V curves, they potentially could have been used as the curve fit was close, so it was a judgment call. Either way, most 6.5” drivers don’t make it past 10V before extreme nonlinearity sets in when the number of voice coil turns in the gap is decreasing as the coil is riding out of the gap.
Following my established protocol for Test Bench testing, I no longer use a single added mass measurement and instead use the physically measured Mmd data (17.2 grams for the BWX-6501). The collected data, in this case the twelve 550 point (0.3V to 15V) sine wave sweeps for each BWX-6501 sample were post-processed and the voltage curves divided by the current curves to generate impedance curves, with the phase derived using the LMS calculation method. I imported the data, along with the accompanying voltage curves, to the LEAP 5 Enclosure Shop software.
Figure 2 shows the 1V free-air impedance curve. I selected the 1V TSL data in the transducer parameter derivation menu in LEAP 5 and created the parameters for the computer box simulations. Table 1 compares the LEAP 5 TSL data and factory parameters for both Bold North BWX-6501 samples.
LEAP LTD and TSL parameter calculation results for the BWX-6501 woofer appear to not correlate well with the factory published data, however the Fs/Qt ratios were close, such than when I programmed in the factory Thiele-Small (T-S) parameters into LEAP, and did a closed-box simulation, the frequency response results were nearly identical. Note that the Bold North sensitivity number is 1W/1m, and is lower than the LEAP's calculated sensitivity at 2.83V. Xmax for the driver, published by MISCO, is actually the XBl number calculated in the Klippel LSI file, which if you have been reading Test Bench reports for a while, is the excursion limit as Bl at rest falls to 82% of its value (the criteria for 10% distortion). Since this is not a calculated Xmax using the driver’s motor geometry, but a reflection of Klippel’s LSI maximum excursion at 10% distortion, I used As I normally do in this column, I followed my established protocol and proceeded to set up computer enclosure simulations using the LEAP TSL parameters for Sample 1.
I programmed two simulated enclosures into the LEAP 5 software, one Butterworth Qtc=0.7 sealed box with 510 in3 air volume with 50% damping material (fiberglass), and a vented Quasi Third-Order Butterworth (QB3) alignment with a 804 in3 volume with 15% damping material. Figure 3 displays the box simulation results for the BWX-6501 woofer with the sealed and vented enclosures at 2.83V and at a voltage level that achieves the 10mm XBl limit. This resulted in a F3 of 63Hz (-6dB=50Hz) with a Qtc=0.69 for the 510 in3 closed box and a -3dB for the vented simulation of 49Hz (-6dB=41Hz).
Increasing the voltage input to the simulations until the Klippel’s LSI XBl limit of the 10mm excursion was reached resulted in 107.5dB at 39V for the sealed enclosure simulation and 110.5dB with a 46V input level for the larger vented box. Figure 4 shows the 2.83V group delay curves. Figure 5 shows the 39V/46V excursion curves.
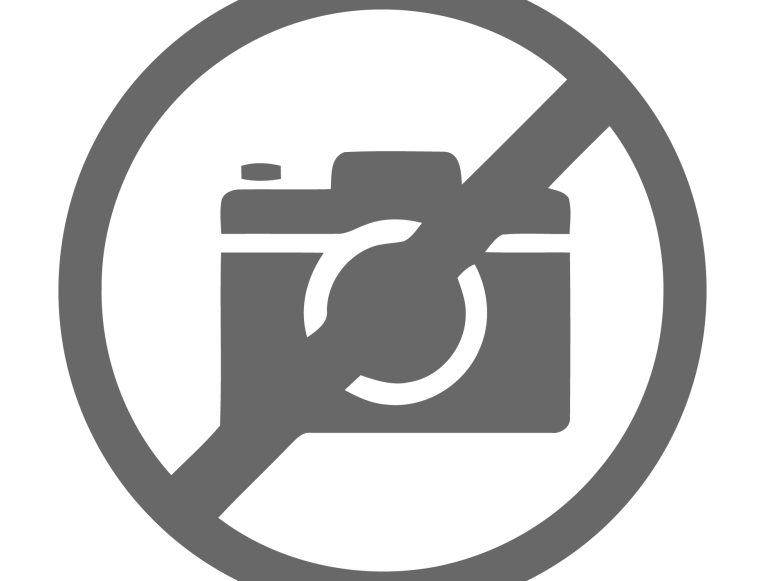
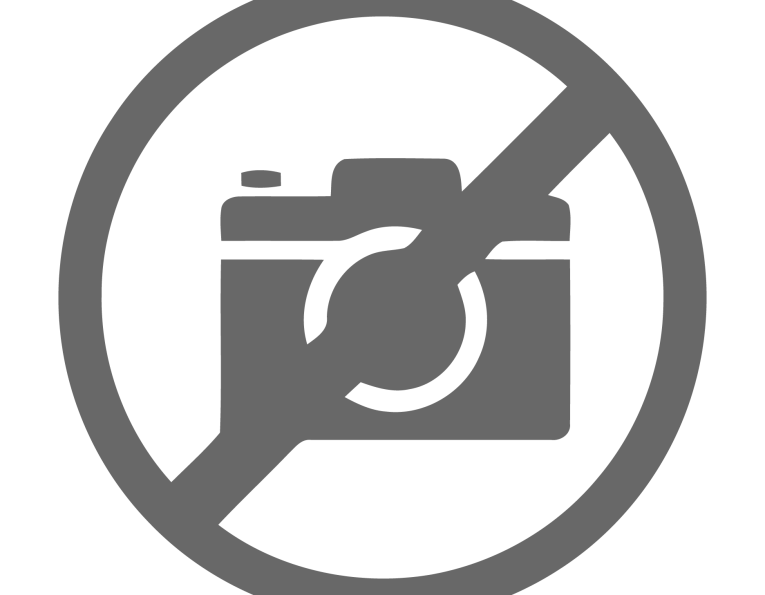

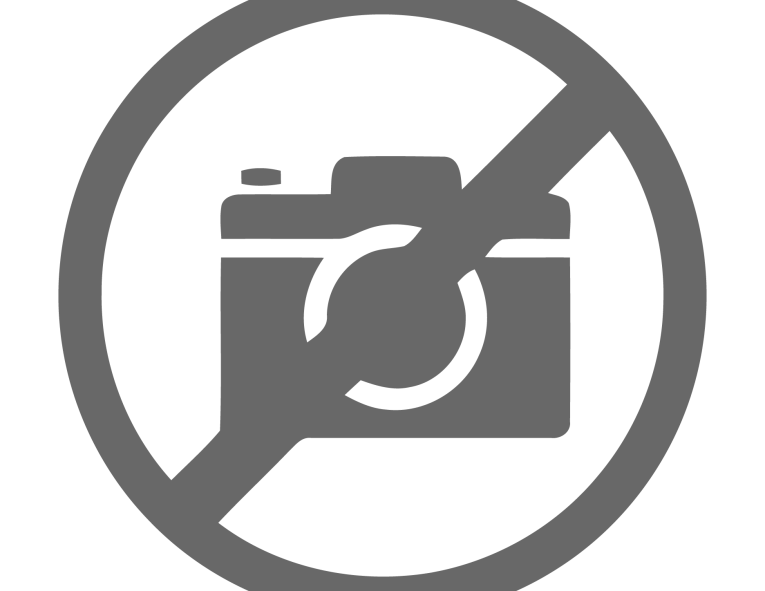
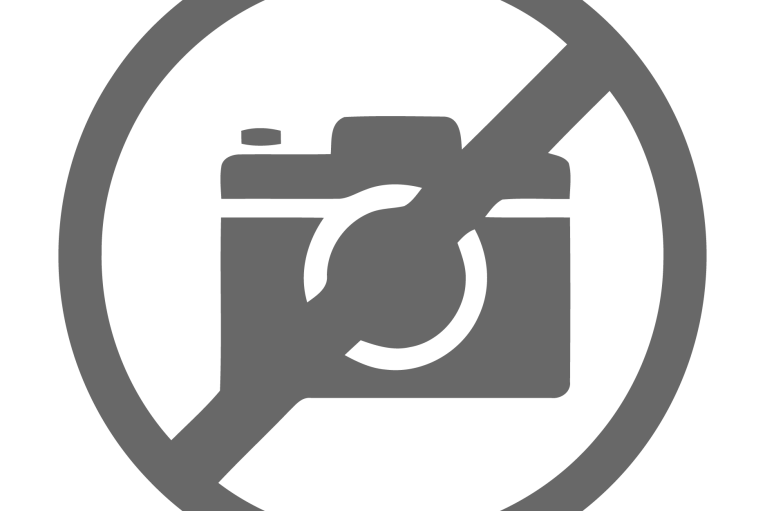
Klippel analysis for the BWX-6501, which this month was performed by Warkwyn (the US purveyor of Klippel GmbH products), produced the Klippel data graphs shown in Figures 6–9. For the near future, I will probably be splitting the Test Bench Klippel analysis between Warkwyn and Redrock Acoustics (Patrick Turnmire).
The Bl(X) curve for BWX-6501 shown in Figure 6 is rather broad, especially for a 6.5” woofer, and with fairly symmetrical behavior with a degree of “tilt” coil-in to coil-out. The Bl symmetry curve in Figure 7 shows a 1.1mm Bl coil-out (forward) offset once you reach an area of reasonable certainty and progressively decreasing to a trivial 0.5mm coil-out offset at the 10mm excursion point.
Figure 8 and Figure 9 show the Kms(X) and Kms symmetry curves for the BWX-6501 driver. The Kms stiffness of compliance curve seen in Figure 8 also reasonably symmetrical and with a degree of rearward (coil-in) offset once the analyzer gets to a position of reasonable certainty (about 4mm). The Kms symmetry range curve shown in Figure 9 exhibits small 0.66mm coil-in (rearward) offset at a region of high certainty that is fairly constant out to nearly 12mm.
Displacement limiting numbers calculated by the Klippel analyzer for the BWX-6501 using the full-range woofer criteria for Bl was XBl at 82% (Bl dropping to 70% of its maximum value) equal to great than 9.94mm for the prescribed 10% distortion level. For the compliance, XC at 75% Cms minimum was only 6.14mm, which means that for the BWX-6501, the compliance is the more limiting factor for getting to the 10% distortion level.
Figure 10 gives the inductance curve Le(X) for this transducer. Motor inductance will typically increase in the rear direction from the zero rest position as the voice coil covers more of the pole in a conventional motor, which is not what you see in the graph. However, the inductive “swing” from maximum inductance to minimum inductance from 10mm coil-in to 10mm coil-out is a very small 0.02mH, which is very good.
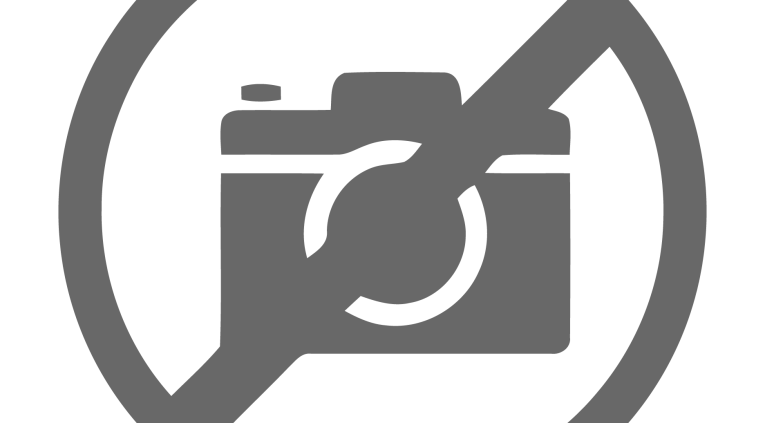
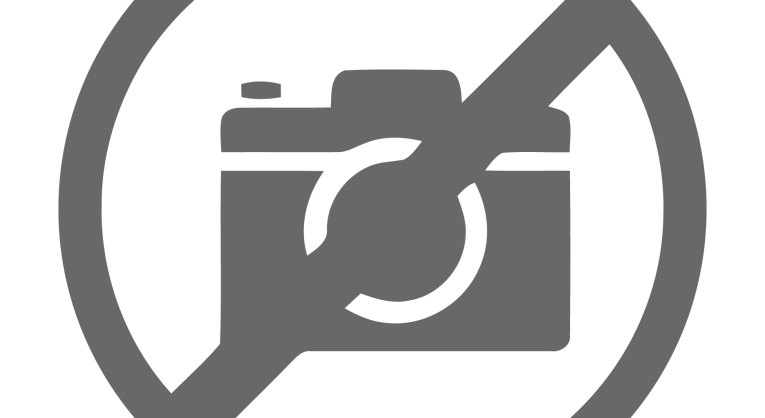
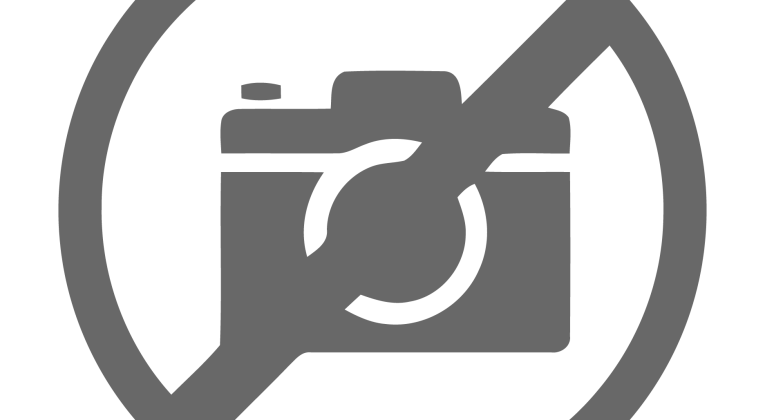
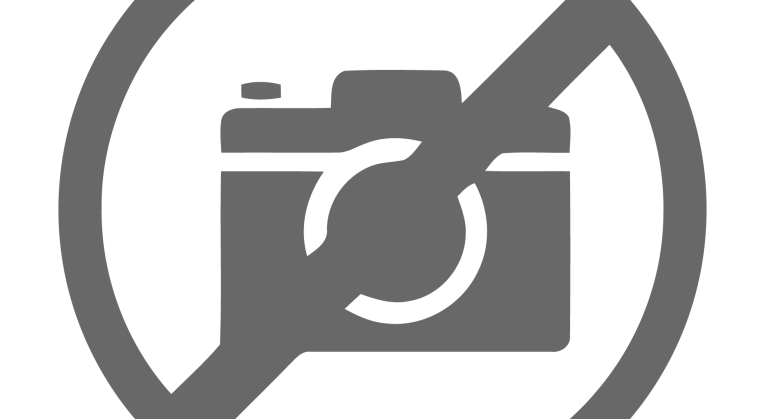
For the remaining test procedures, I mounted the BWX-6501 in a foam-filled enclosure that had a 17"×8" baffle and then measured the device under test (DUT) using the LoudSoft FINE R+D analyzer and the GRAS 46BE microphone (courtesy of LoudSoft and GRAS Sound & Vibration) both on- and off-axis from 200Hz to 20kHz at 2.0V/0.5m, normalized to 2.83V/1m, using the cosine windowed Fast Fourier Transform (FFT) method. All of these SPL measurements also included a 1/6 octave smoothing.
Figure 11 gives the BWX-6501’s on-axis response, indicating a fairly smooth rising response with no break-up modes or peaking out to about 3kHz. However, there is a peak in the response at 4kHz, where the driver begins its low-pass roll-off. Figure 12 displays the on- and off-axis frequency response at 0°, 15°, 30°, and 45°, -3dB at 30°, with respect to the on-axis curve occurs at 3.2kHz, so a cross point in that vicinity should work well to achieve a good power response. Figure 13 gives the normalized version of Figure 12. Figure 14 displays the CLIO horizontal polar plot (in 10° increments) for the BWX-6501 woofer. And finally, Figure 15 gives the two-sample SPL comparisons, showing a close match (less than 0.5dB) to about 2.5kHz, with some variation above that which will be in the stop-band of a low-pass filter.
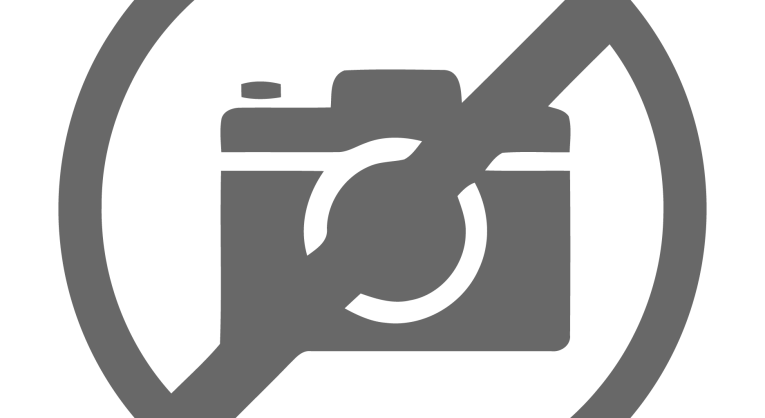
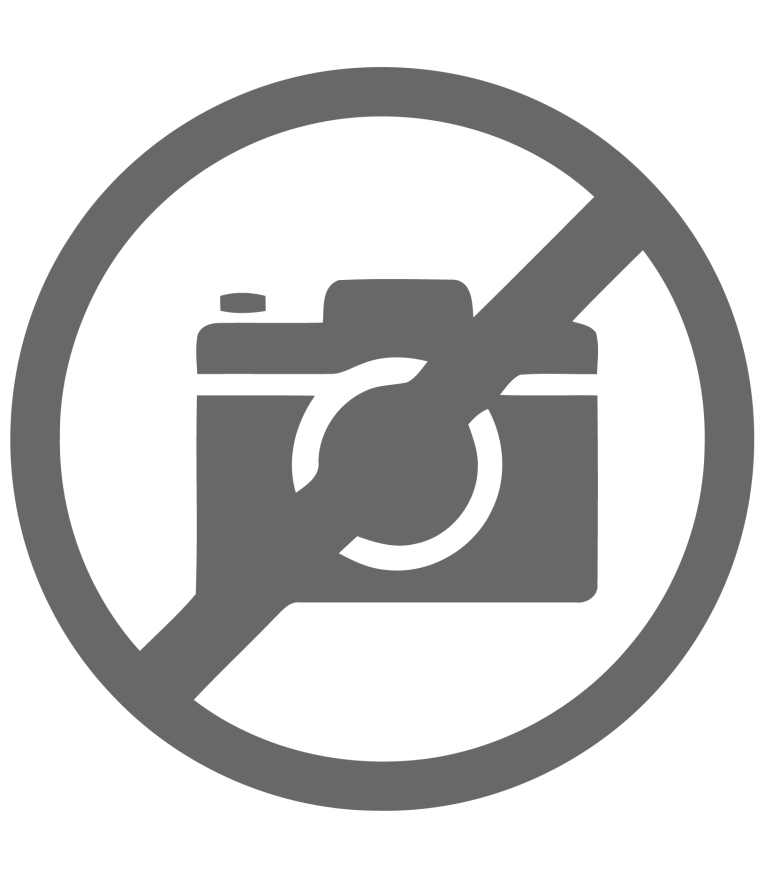

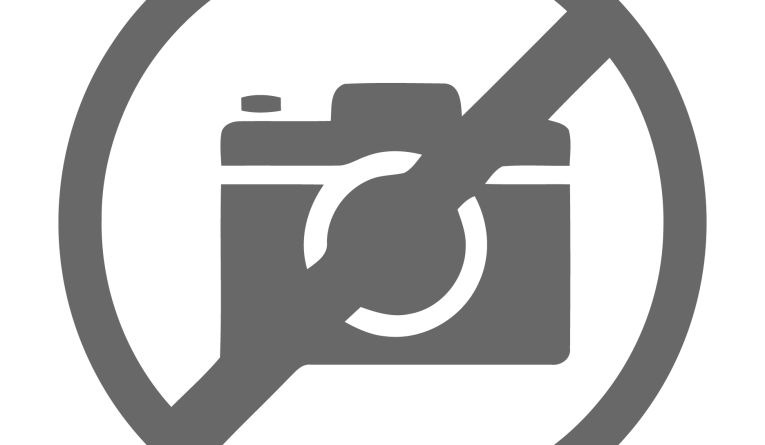
For the remaining series of tests on the BWX-6501, I employed the Listen, Inc. SoundCheck AudioConnect analyzer and SCM microphone (graciously supplied to Voice Coil by the folks at Listen, Inc.) to measure distortion and generate time-frequency plots. For the distortion measurement, I mounted the BWX-650 rigidly in free-air, and set the SPL to 94dB (my criteria for home audio transducers) at 1m (6.16V) using a SoundCheck pink noise stimulus. Then, I measured the distortion with the Listen microphone placed 10cm from the driver. This produced the distortion curves shown in Figure 16.
I then employed the SoundCheck software to get a 2.83V/1m impulse response and imported the data into Listen’s SoundMap Time/Frequency software. Figure 17 shows the resulting cumulative spectral decay (CSD) waterfall plot. Figure 18 shows the Wigner-Ville plot (chosen for its better low-frequency performance).
Looking at all the various data I collected for the new Bold North Audio BWX-6501 6.5” midbass driver and considering its BLX2 high excursion capability, as well the overall design and build quality, this is a really well-crafted product for the high-end hi-fi market. For more information, visit www.miscospeakers.com/product-lines/bold-north-audio. VC
This article was originally published in Voice Coil, December 2020.