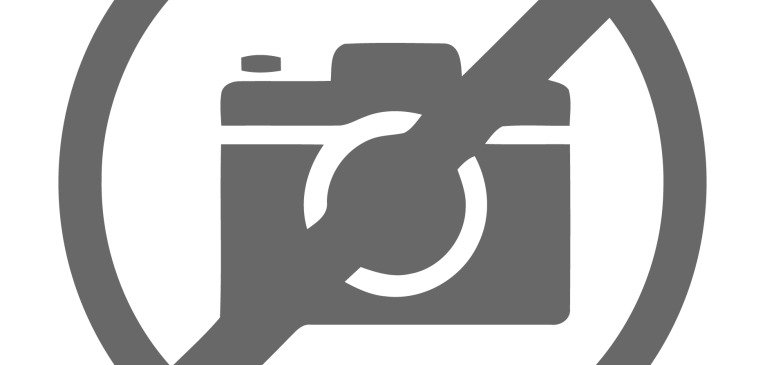
Principle of Operation
In Fig. 1, the open circuit voltage gain is provided by the grounded emitter stage formed by Q1 and R2. This is followed by a unity-voltage-gain emitter-follower stage formed by Q2 and R4. R3 provides negative feedback to better define the gain, improve linearity, provide DC C2 rolls off the open loop gain above audio frequencies to prevent oscillation.
It also attenuates any radio frequencies picked up (see “Effects of Nonlinearities” section). C1 and C3 provide DC isolation at the input and output, respectively. C4 reduces power rail excursions.
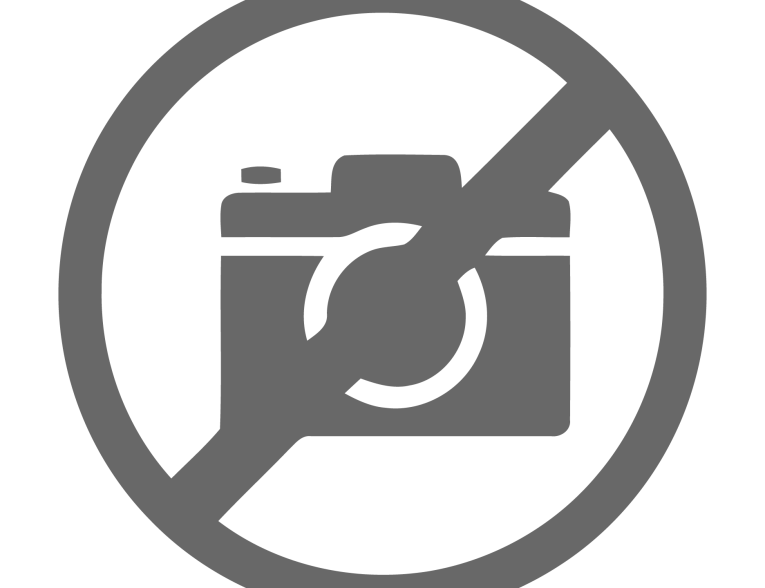
DC Bias
Q1 base is one diode drop (about 0.6V) above ground, drawing current from Q2 emitter through R3, across which there is a negligible DC voltage. Q2 base is therefore about 1.2V above ground. Taking into account the 2.2kΩ in the soundcard between the ring and the 5V supply, the ring settles at about 3.5V.
Open Loop Gain.
The DC voltage across R2 is 3.5-1.2 = 1.3, so at room temperature the voltage gain of the grounded emitter stage is about 40 × 1.3 = 52.
Closed Loop Gain.
The model for calculating the closed-loop gain (Fig. 2) contains:
• An ideal amplifier of gain -A representing the gain of the grounded-emitter stage with A = 52.
• Resistance x representing R1 in series with the microphone impedance; the impedance of an SM10A is 223Ω, so x = 323Ω.
• Resistance z represents the input impedance of the grounded-emitter stage.
This is β × the emitter impedance, which is R2/A. The lower bound on β is 270, giving a lower bound on z of 29kΩ.
• Resistance y represents R3 (10kΩ).
Analysis of this circuit gives the closed loop gain as: -Ayz/xy + yz + (1 + A)zx
Substituting for A, x, y, and z gives a (negative) closed-loop gain of 19.
Nonlinearities
The emitter impedance of a bipolar transistor is inversely proportional to the emitter current. This results in two sources of nonlinear gain:
1. The gain of the grounded emitter stage.
2. The output impedance of the emitter follower, which results in nonlinear gain depending on how the output is loaded.
The Grounded -Emitter Stage
The gain of a common emitter stage is proportional to the emitter current and therefore proportional to the voltage drop across R2. The gain when the output signal is high is less than when the output signal is low. Assuming a 1mV signal from the microphone, the signal is about 20mV at Q1 collector, which means that the open loop gain can vary by almost 2%. However, the negative feedback limits the variation of the closed loop gain to about 0.7%.
The Emitter -Follower Stage
The output impedance of the emitter-follower stage is inversely proportional to Q2 emitter current. With a 20mV signal, this can vary by about 6% around about 200Ω. However, the soundcard microphone input impedance is about 2.2kΩ, so the effect on open-loop gain is only about 0.3%. The effect on closed-loop gain is only about 0.1%. In addition, the nonlinearity is opposite to that of the grounded emitter stage, so it subtracts from the effect due to the grounded emitter stage.
Capacitively loading the output can introduce more nonlinear distortion. However, a significant effect would require several nanofarads of capacitance, much more than any likely parasitic.
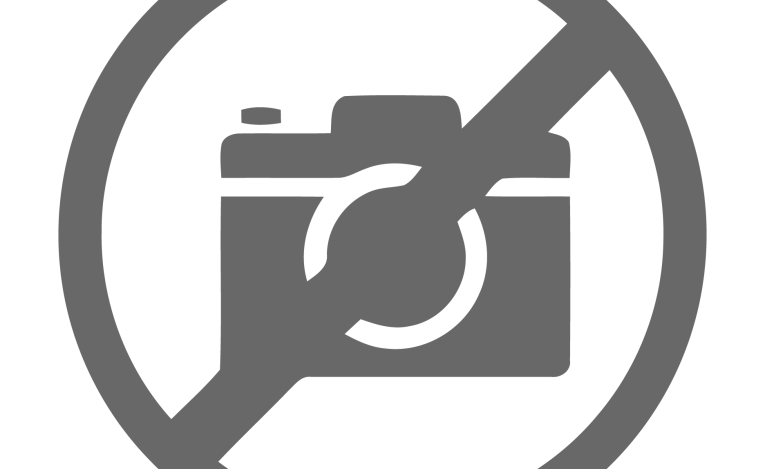
Effects of Nonlinearities
For variation of gain, as a function of signal level introduces two components to the signal:
1. Frequencies double that of the original (0.6% variation of gain introduces 0.15% second harmonic distortion). Given the high harmonic content of human speech, this is unlikely to adversely affect speech recognition.
2. A DC offset proportional to the square of the signal level. At first sight this may seem inconsequential, but it turns the amplifier into a radio receiver unless you take steps to attenuate radio frequencies1.
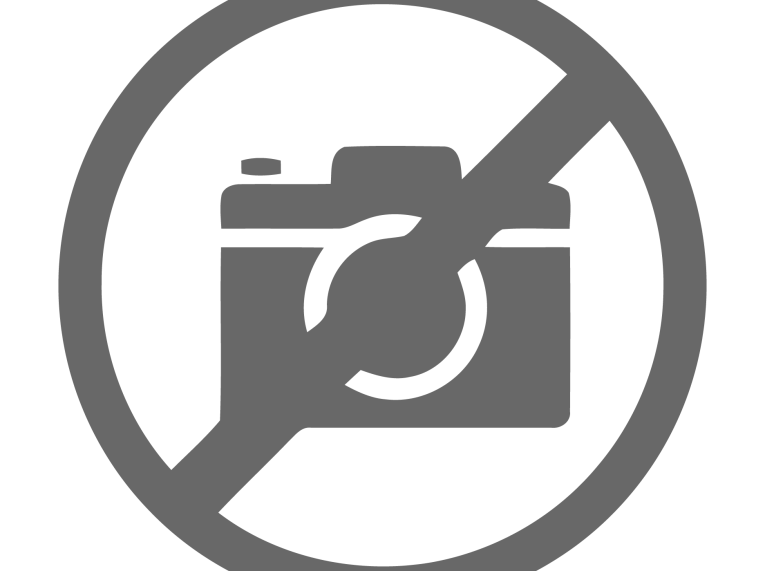
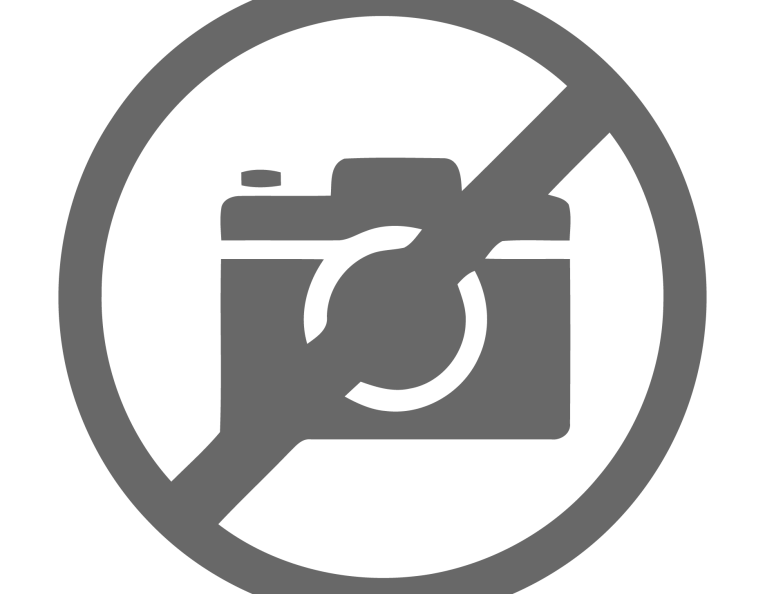
Mechanical Design
The printed circuit design is shown in Fig. 3. You can use Radio Shack’s smallest project box to house the amplifier. It has a convenient stud in the middle which provides a press-fit mount for a 0.1″ pitch perforated board with one hole drilled out to 5mm. Drill holes for the cables in each end with the box clamped tightly closed.
Then insert the board, cables attached, into the open box (Photo 1), close the box, and secure it with a single self-tapping screw. The complete assembly with cables and connectors is shown in Photo 2.
Footnote
1. The first version of this amplifier rolled off the loop gain using a capacitor to the collector of Q1. When conditions were right it happily picked up KGO at 810kHz AM, causing great confusion to the speech recognition. aX
Parts List
C1 tantalum capacitor | 10μF | 16V |
C2 ceramic capacitor | 47nF | 50V |
C3 tantalum capacitor | 10μF | 16V |
C4 tantalum capacitor | 10μF | 16V |
R1 carbon film resistor | 100Ω | 0.25W |
R2 carbon film resistor | 5.6kΩ | 0.25W |
R3 carbon film resistor | 10kΩ | 0.25W |
R4 carbon film resistor | 2.2kΩ | 0.25W |
Q1 silicon NPN transistor | NTE16 | |
Q2 silicon NPN transistor | NTE16 |
This article was originally published in audioXpress, February 2008.
About the Author
Paul Loewenstein has a BA in 1971 from the University of Cambridge, MA in 1974, and Ph. D in 1994. His early career at Schlumberger in the UK and France involved analog systems from high-frequency IC amplifiers, vibrations of thin shells, and transients on high-voltage power lines. His professional activity has been purely digital since his move to the US in 1981, working for Schlumberger, National Semiconductor, Mitsubishi, and Sun Microsystems.