Conventional wisdom holds that a pure voltage source amplifier is ideal for audio applications, and generally designers of loudspeakers work to that assumption. This belief has particularly been dominant since the development of high power solid-state amplifiers in the 1960s. A small minority of audiophiles thinks otherwise, and these people often use low wattage tube amplifiers with unusual-looking speakers. Well, of course entertainment is full of fringe elements.
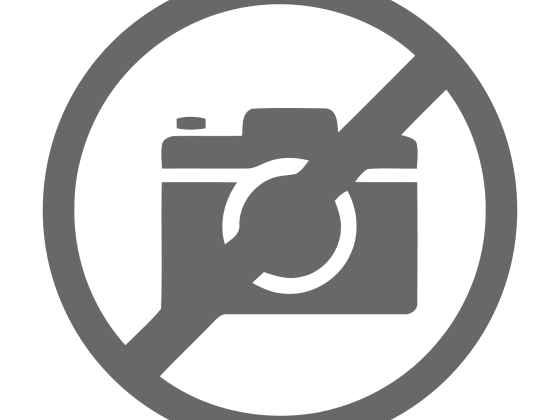
A couple of years ago Kent English and I were playing around with various ribbon tweeters, noting that without a matching transformer the ribbons themselves seemed perfectly happy driven by a current source. We built an audio current source that delivered 1A of AC current per input volt to a maximum of 10A. This kind of amplifier is known as a power transconductance amplifier.
Such circuits are fairly common as small chips, but those do not have the high current needed to drive a loudspeaker. The amplifier had a high output impedance, and thus no damping factor, but we found that the ribbon in the tweeter didn’t seem to need a damping factor, measuring and sounding a bit
better without the matching transformer. We decided that a current source was likely delivering more accurate force/acceleration to the ribbon than a voltage source, and after playing with it for a couple of days we put it away and went on to other projects.
Also about this time Kent and I began playing with full-range drivers and loudspeakers that deliver bass, midrange, and treble with one cone. In a number of ways they don’t measure as well as multiple specialized drivers, particularly at the bottom and the top, but there is something aesthetically appealing about the simplicity of the idea, and on many occasions they manage to sound very good, especially driven by tube amplifiers.
Most interesting are the full-range high-efficiency drivers that deliver the goods with only a watt or so. It’s a big challenge to a designer to deliver a good-sounding full-range acoustic transducer with 100dB/watt efficiency. When it is well achieved, you get a wealth of detail, exceptional dynamic range, and a sense of musical “liveliness” that you don’t often hear elsewhere.
Tube amplifiers seem to bring out the best in such drivers. They have more bottom end, a warmer, mellower mid and upper mid-range, and often more top octave. By comparison, the “best” solid-state amplifiers make them sound more like transistor radios - less bottom and an occasionally strident upper midrange. If you are a solid-state kind of guy (like me), you start wondering how that could be, and if you are a tube aficionado, you smirk and say, “I told you so.” The solid-state guy probably starts fixing the response with a parametric equalizer, and the tube guy enjoys his music with a nice glass of wine.
Critical damping - that resistive combination of electrical source impedance, suspension friction, and acoustic load - occurs when you apply a step pulse to the voice coil and the cone’s motion doesn’t overshoot. Under-damping results in bass notes that hang around a little longer than the amplifier intended. Over-damping has good bass transient control, but also significant loss of bottom end response. Generally we want something in between - something near to critical damping, and whether we under-damp or over-damp slightly seems to be a matter of taste.
The need for electrical damping is different for each type of loudspeaker and acoustic environment. High-efficiency full-range drivers are more easily damped than other types due to powerful, efficient motors and light cones. Looking at their bass response curves, we conclude that they are easily overdamped, resulting in excessive loss of bottom end. This partly explains the preference for tube amps with such loudspeakers.
Anyway, this assortment of observations arrived at a confluence when I hooked up a Son of Zen amplifier (Audio Electronics 2/97) to a pair of Fostex 208Es in sealed enclosures. The Son of Zen operates without feedback and has an output impedance of about 16Ω, which would be a damping factor of 0.5 - minuscule compared to the 100 to 1000 you can get with regular solid state amplifiers.
With the low damping factor it was a totally different speaker. It suddenly had bottom end response and a better top end. It still had the same annoying upper-midrange that made Dick Olsher (www.blackdahlia.com) devise his passive equalization network. The low damping factor didn’t cure the upper midrange faults, but it seemed to work improvements everywhere else.
A year later, Kent has acquired about 20 different full-range/ mid- and high-efficiency drivers for us to play with (it’s part of his job description). We’ve spent as much time exploring them as we reasonably can, trying different things to coax the best sound out of them with a current source amplifier and various passive parallel networks.
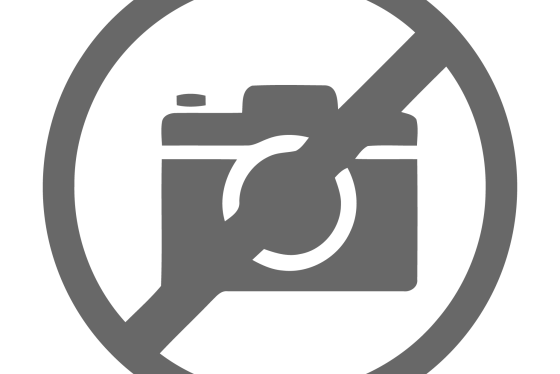
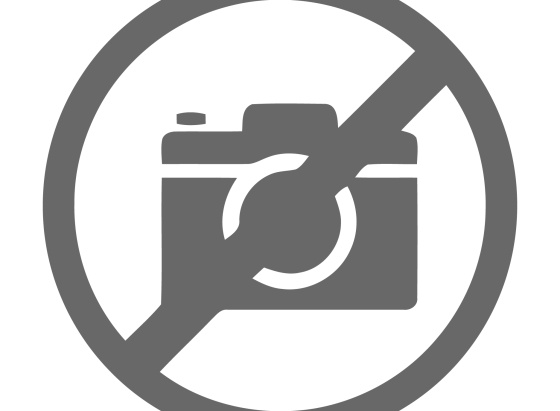
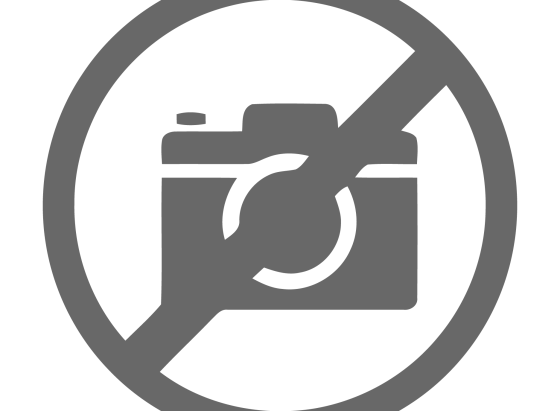
What Is A High-Efficency/Fullrange Loudspeaker?
To get high-efficiency and wide bandwidth, we fundamentally need two things - a great motor and a great radiating surface. A great motor means getting the most force/acceleration for the least amount of electricity. It means a big magnet with lots of magnetic density in a precisely machined gap where a very light voice coil sits (precisely). This voice coil is wound in a cylindrical assembly that maximizes the current exposed to the magnetic field and generates the most amount of force (acceleration) for a given amount of electrical current - in other words, an expensive motor.
The other half of the equation is the cone assembly attached to the voice coil. Here we start seeing more of the art that is involved in such a speaker, in contrast to the fine motor engineering.
The radiating surface for such a loudspeaker must be very light, to maximize the acceleration from the voice coil. At the same time, the cone needs to be stiff and inflexible, so that this acceleration can be accurately transmitted to the entire radiating surface at once. When this fails at high frequencies (which it will), the cone needs to decouple the force gracefully to a smaller and smaller surface so that it becomes effectively smaller at the highest frequencies. In practice it’s a lot of art coupled with even more trial and error. Thomas Edison could have made a fantastic version of such a speaker, and for his time I believe he did.
Besides obviously requiring low power and only one driver per channel, the advantages of a full-range efficient loudspeaker are found in three characteristics. First, the speaker requires no crossover network to apportion frequency bands to different drivers, and does not suffer the phase shifts that come with such filters. Second, the sound radiates from one source, so that diffraction effects between drivers are removed. Third, the electrical and mechanical qualities required by such drivers give rise to subjectively good dynamic range and detail.
This is assuming an ideal example of a full-range efficient driver. The reality can fall short of this promise. Real drivers tend to be too limited to satisfactorily deliver both the top and bottom octaves of audio. The decoupling of high frequencies on the cone is often not smooth, and with that comes response peaks and dips in the upper midrange, often followed by a high-frequency response rolloff.
On the bottom end, over-damping of the very light cone contributes to a rolloff starting at an octave or more above resonance, so that many examples of such drivers fall short below 100Hz - in a closed box with a voltage source their response is as much as 15dB down at resonance. Some of these drivers are better than others, most of them are delicate, and some of them are very expensive.
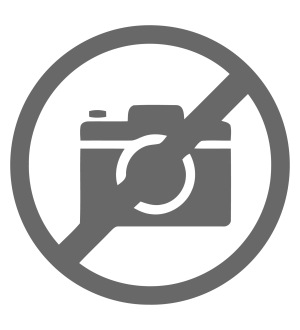
Power Transconductance Amplifiers
This is a fancy name for a power current source. An input voltage causes the amplifier to deliver a proportional output current. Of course, this sort of thing would occur with an ordinary amplifier driving a pure resistance, but a loudspeaker circuit is not pure resistance. It possesses numerous reactive elements, some due to inductance and capacitance in the electrical circuit, and some from the reaction to motion of the voice coil in a complex mechanical system. Fed by a voltage amplifier, the current through the loudspeaker voice coil is not directly or instantly proportional to the input to the amplifier.
Ordinarily, loudspeakers are designed around this assumption, but the “piston model” of loudspeakers presumes that over a specific range the acoustic output mirrors the acceleration of the voice coil/cone assembly, and this is reflected by the current through the voice coil. The most precise way to develop that specific current is with a current source amplifier. Such an amplifier ignores the impedances in series with the circuit, the resistance and inductance of the wire and voice coil and the back electromotive force produced by the cone motion.
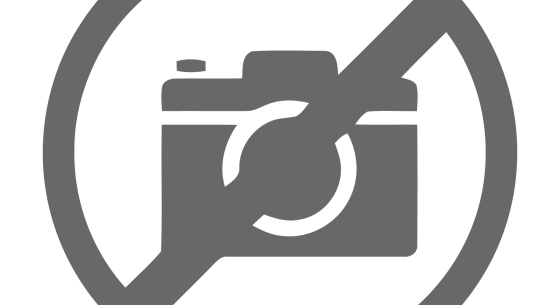
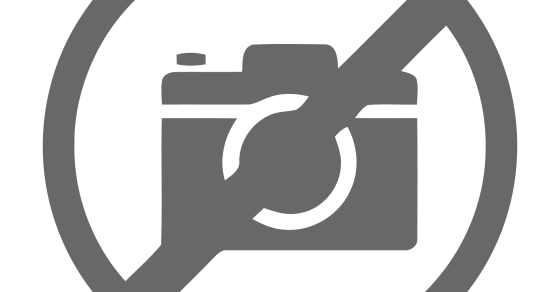
As I said, most speakers are designed around voltage sources, but there are few instances where a current source can be used to advantage. One of the best ones is the category of full-range high-efficiency drivers. Why is that? First, such drivers are able to take advantage of acoustic and suspension resistance to achieve some or all of the damping that they need to prevent excessive overhang because their moving mass is very light. With their efficient motors, even a high source impedance is often enough to give critical damping.
Second, their impedance curve tends to reflect their needs - more current at the low-frequency resonance and at the high end, both areas where the output response has fallen off with increased speaker impedance. If you want, the current through the voice coil can be made constant regardless of the variations in the acoustic environment. The voice coil force is invariant whether the cone is loaded into a horn, sealed box, bass reflex, or whatever else you care to mount it in.
Third, they are very easy to adjust in the upper midrange and high-end where you typically run into peaking problems. Most of these drivers are too hot in the upper midrange, and quite a few of them fail to make it to 20kHz. With a simple parallel network, you can arrange for a midrange dip followed by an increase at the top, evening the speaker response.
More often on the higher quality drivers, you simply want an equalized “shelf” where everything above a specific frequency is evenly attenuated. The adjustments for damping at the low end and the mid/high peaking is easily accomplished using R, RC, or RLC (resistor/inductor/capacitor) networks wired in parallel with the output of the amp.
You occasionally see this sort of equalization with full-range high-efficiency drivers with voltage source amplifiers, but there the elements are paralleled and then placed in series with the driver. With a current source, the equivalent circuit is the elements placed in series and then paralleled with the driver.
Of course, there’s the added benefit that the resistive element of the speaker cable and the connection points can be made to largely disappear with a current source. To obtain the best effect, the loading network is placed close to the driver instead of the amplifier.
The following pages will show what we found with a variety of full-range sensitive loudspeaker drivers when we drove them with an active current source and played with parallel networks to shape the response to our liking. Most of the examples we will examine do not require true current source amplifiers, only amplifiers of quite high output impedances. Most of these cases will be happy with approximately 47Ω or so output impedance, and prefer 47Ω loaded in parallel with the output of a current source. That being the case, you can build a Thevenin equivalent of such a current source by placing a large resistor (later here to be known as R0) in series with the output of about a high wattage voltage source amplifier, and get something similar.
I’m not saying it will equal a spiffy First Watt F1 (being Class A and no feedback and all), and your resistor will run hot. On the other hand, you probably already have such a voltage source amplifier, and some of these speakers are quite cheap, allowing you a taste of these forbidden pleasures without high expense.
I want to emphasize that this does not serve as any sort of comprehensive guide to designing systems around this approach. You can build up the examples, and they will probably measure about the same, but remember that mostly we are restricting ourselves to the sealed box case for simplicity, and most of the time in the real world they are used in an enclosure that also uses the back wave of the driver. Also, the best measured curve is often not the best-sounding one, so be prepared to try various values to get satisfactory sound.
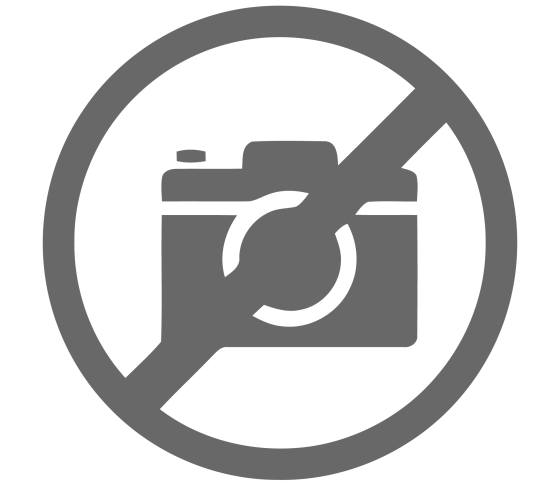
Amplifier/Loudspeaker/EQ Network Model
Figure 1 shows a current source amplifier, the simplified model of the loudspeaker’s impedance, and the generalized network that we can parallel with the speaker to enhance its performance. The speaker model consists of L2/R2/C2 that model the fundamental resonance, and R3/L3 that give us its DC resistance and high-frequency inductance.
A good example of this is the simulation of the Pioneer b20fu20–51 driver. The values L2 = 0.05H, R2 = 27Ω, C2 = 300μF, R3 = 7Ω, and L3 = 0.1mH give a reasonable facsimile of the measured free-air impedance curve in Fig. 2. Unless otherwise noted in a discussion, we will be addressing the driver mounted in a sealed box of a given volume, as it is the easiest design to consider. Horns and rear-loaded acoustic systems are more complicated to simulate and measure, and the test results are more difficult to duplicate and interpret.
One advantage of a sealed box for full-range drivers is that the excursion is more limited below resonance, which can reduce distortion, particularly at higher levels. Driven by a voltage source, we usually see anywhere from −6 to −15dB response loss at the low-frequency resonance, and a top end which increases or declines (or both) depending mostly on the cone construction and voice-coil inductance. Driven by a current source, we note that the bottom end is bumped up at resonance and the top end is increased over the performance of the voltage source.
Assume that we want to make the best frequency-response curve for a given system. This is not necessarily the best-sounding system possible, but is subject to less argument. We can trim the damping “Q” of the low-frequency rolloff knee by trying different values for R0 (L0 = 0 in this case) until it flattens out to our taste. Most of the drivers we worked with were happy 47W. It is surprising that so little damping gave such dramatic results, but as previously pointed out, this category of loudspeaker responds well to small amounts of damping.
Usually we also want to adjust the upper midrange and high end. Often these drivers will show an upper-mid peak followed by a decline before the top end is reached. We can compensate for this by our selection of R1, C1, and L1, which can be used either to produce a shelf or a dip to flatten out the response.
With these models in mind, we measured all the sample speakers of interest, developing networks to optimally flatten out their response with a current source, and comparing this with the same speaker driven by a voltage source. We used a Vidsonics “virtual crossover” substitution box to play with the parallel loading and took both near field (an inch or so) and far field (1 meter) response measurements using Mlssa.
Again, the results here are not necessarily the best-sounding that you can get, and I am not attempting to address that as such, given the wide range of source material, listening environments, and subjective taste. Typically these exact network figures are not subjectively preferred. Usually this is because listeners tend to prefer a higher value for R1 than in Table 1.
If you decide to use one of these networks, consider the possibility that R1 is the minimum value and use a switch or high power (5W) potentiometer to variably increase the value to as much as twice R1. Less often, you might consider a different value for L1 in trimming the top end.
I repeat: Consider this as an experimental guide and a good starting point. Be prepared to change the values to suit your needs, and remember that these figures were obtained in stuffed
sealed enclosures in a large room out from the wall. Your results will certainly vary. Also remember my comments reflect offhand reactions. Please do not treat them as reviews.
Table 1 shows the values that resulted in improved frequency-response curves with current sources for different speakers in different-size boxes.
Now that you have an overview of the interface network, let’s look at a few individual drivers in more detail.
(Continues on the next page)
www.firstwatt.com | passlabs.com | www.passdiy.com
This article was originally published in audioXpress, October 2004