Currently, some of the most popular transducers in consumer electronics are the 2”–3.5” diameter full-range drivers (e.g., Scan-Speak’s 2” 5F/8422T01 featured in Voice Coil’s October 2013 issue). These mini woofers are being extensively used in TV soundbars, pedestal soundbars (soundbars that contain down-firing subwoofers that double as a TV stand), docking stations, Bluetooth-powered speakers, and desktop speakers (e.g., the Sonos products, Jambox, Dr. Dre., Samsung, Bose, etc.). If you are an OEM driver manufacturer, it seems like a good category to choose.
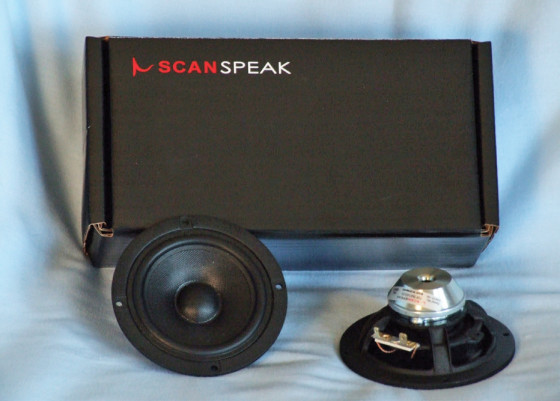
To this end, Scan-Speak set out to make the one of the best 3.5” full-range drivers on the market. I think the company accomplished its goal with the new 10F/8414G10. Scan-Speak’s 10F/8414G10 is built on a proprietary castaluminum frame that is fully vented below the spider mounting shelf for enhanced cooling (see Photo 1). The cone assembly consists of a black patented NRSC fiberglass cone that has a rear-damping coating applied plus a fiberglass dust cap, suspended with a SBR surround and a black-cloth flat spider (damper). For a 3.5” driver, the 10F/8414G10 has a fairly standard 19.4-mm diameter voice coil wound with round copper wire on a titanium former, terminated to a set of gold-plated solderable terminals.
Driving the cone assembly is a neodymium motor using a neodymium ring magnet rather than a slug, and a polished milled return cup, plus a copper cap Faraday shield on the pole piece for distortion reduction. Additional cooling is provided by a 7-mm diameter pole vent. The 10F/8414G10’s literature notes the frame is made in Denmark.
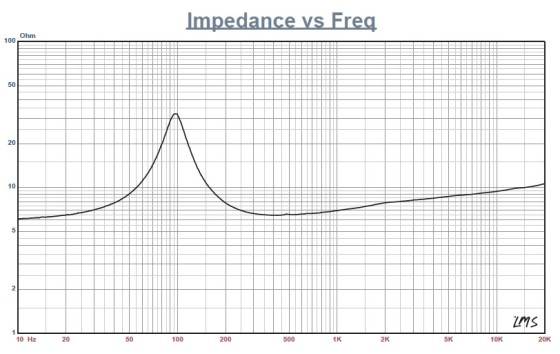
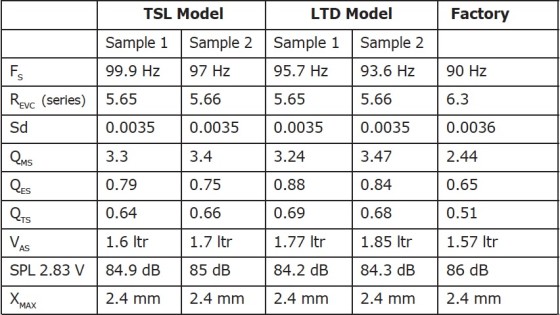
I commenced testing the 10F/8414G10 full-range driver using the LinearX LMS analyzer and VIBox to create voltage and admittance (current) curves. I clamped the driver to a rigid test fixture in free air at 0.3, 1, 3, 6 and 10 V, with the 10-V curves discarded as being too nonlinear for LEAP 5 to get a good curve fit. Again, I no longer use a single added mass measurement instead I used the manufacturer’s measured Mmd data.
Next, I post-processed the four 550-point stepped sine wave sweeps for each of the 10F/8414G10 samples and divided the voltage curves by the current curves (admittance) to produce the impedance curves, phase generated by the LMS calculation method. I imported them, along with the accompanying voltage curves, to the LEAP 5 Enclosure Shop software. Since most T-S data provided by OEM manufacturers is produced using either a standard method or the LEAP 4 TSL model, I used the 1-V free-air curves to also create a LEAP 4 TSL model.
Figure 1 shows the 1-V free-air impedance curve. I selected the complete data set, the multiple voltage impedance curves for the LTD model, and the 1-V impedance curve for the TSL model in the LEAP 5’s transducer derivation menu and created the parameters for the computer box simulations. Table 1 compares the LEAP 5 LTD and TSL data with factory parameters for both of the Scan-Speak 10F/8414G10 samples.
LEAP TSL parameter calculation results for the 10F/8414G10 full-range driver were close to the factory data, while the QTS for the LTD multi-voltage parameters exhibited a somewhat higher number. However, I followed my usual protocol and used the LEAP LTD parameters for Sample 1 to set up the computer enclosure simulations. I programmed two computer enclosure simulations into LEAP, a 425-in3 sealed box alignment (50% damping material in the box) and a 270-in3 Chebychev/Butterworth-type vented alignment tuned to 64 Hz (15% damping material).
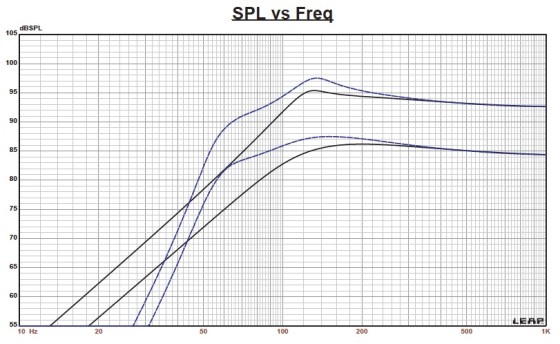
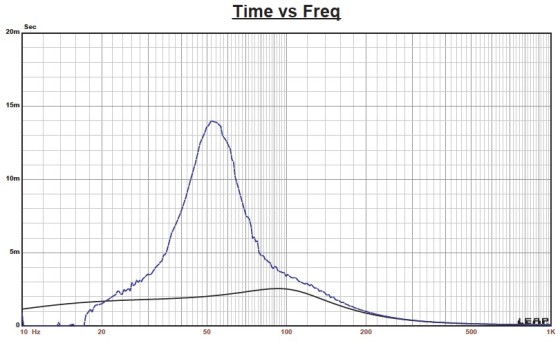
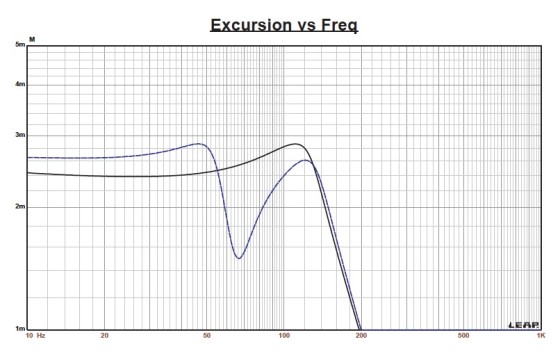
Figure 2 shows the results for the 10F/8414G10 in the two box simulations at 2.83 V and at a voltage level sufficiently high enough to increase cone excursion to 2.8 mm (XMAX + 15%). This produced a F3 frequency of 104 Hz with a box/driver 0.77 QTC for the 425-in3 sealed enclosure and –3 dB = 82 Hz for the 270-in3 vented simulation.
Increasing the voltage input to the simulations until the maximum linear cone excursion was reached resulted in 95.3 dB at 9 V for the sealed enclosure simulation and 97.5 dB with the same 9-V input level for the vented enclosure. Figure 3 and Figure 4 show for the 2.83-V group delay curves and the 9-V excursion curves).
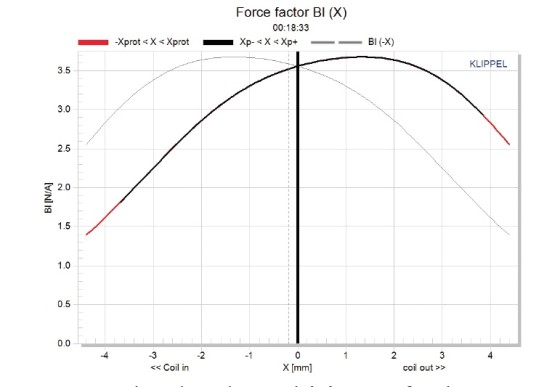
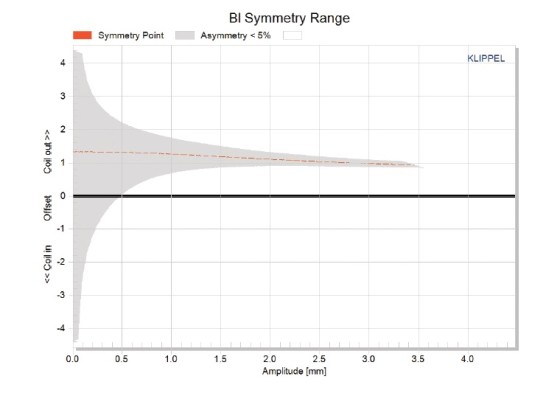
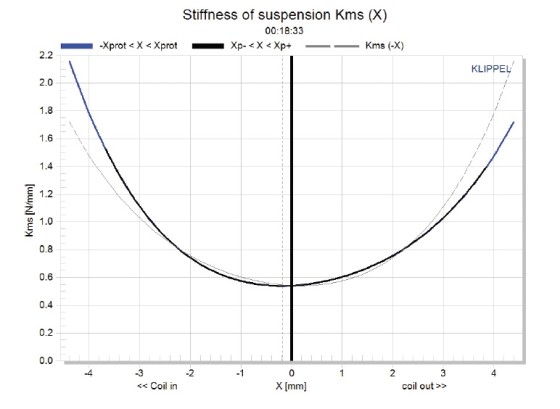
Turnmire also performed the testing on the 10F/8414G10, which produced the Bl(X), KMS (X) and Bl and KMS symmetry range plots shown in Figures 5–8. The Bl(X) curve for the 10F/8414G10 shows some obvious asymmetry (see Figure 5). Looking at the Bl symmetry plot, it appears this is mostly a matter of the voice coil not being located at magnetic center and most likely at the gap’s physical center, which are often not coincidence (see Figure 6).
Figure 7 and Figure 8 show the KMS(X) and KMS symmetry range curves for the 10F/8414G10. The KMS (X) curve is rather symmetrical, and has a minor rearward (coil-in) offset of less than 0.18 mm at the rest position, decreasing to an insignificant 0.05 mm at the physical XMAX position. The 10F/8414G10’s displacement limiting numbers were XBl at 82% (Bl is 1.9 mm) and for crossover at 75%, CMS minimum was 1.8 mm. For the 10F/8414G10, compliance is the most limiting factor for prescribed distortion level of 10%.
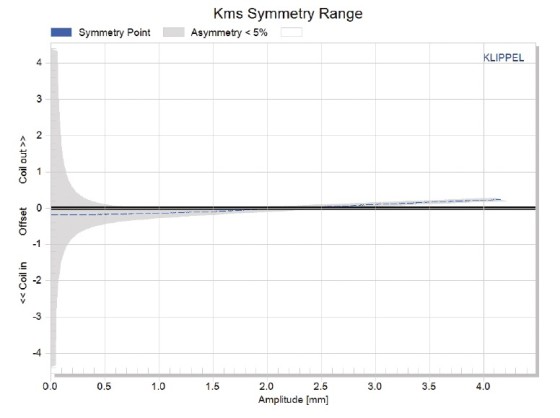
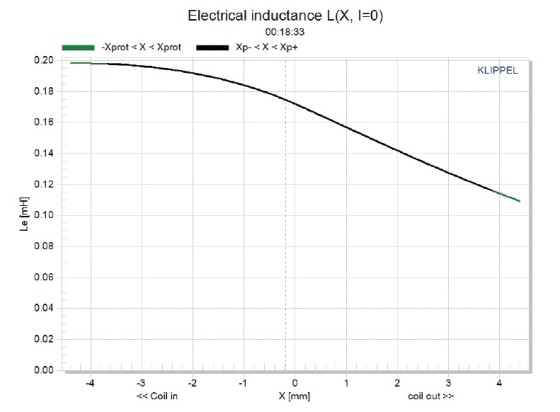
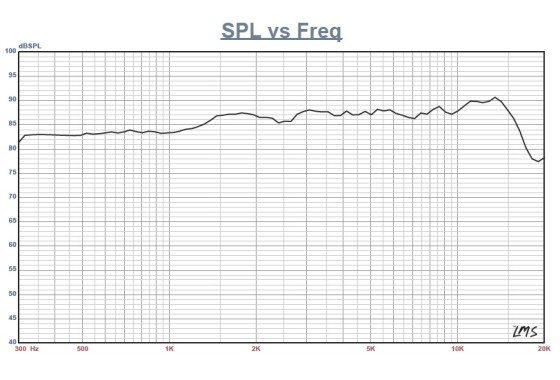
Figure 9 shows the inductance curve Le(X) for the 10F/8414G10. Inductance will typically increase in the rear direction from the zero rest position as the voice coil covers more pole area, which is what happens. However, the inductance variation is only 0.06 mH from the in and out XMAX positions, which is very good and mostly due to the copper cap.
Next I mounted the 10F/8414G10 in an enclosure which had a 5” × 10” baffle. I filled the baffle with damping material (foam) and used the LinearX LMS analyzer set to a 100-point gated sine wave sweep. Then, I measured the transducer on- and off-axis from 300-Hz to 40-kHz frequency response at 2.83 V/1 m. Figure 10 shows the 10F/8414G10’s on-axis response indicating a smoothly rising response to about 15 kHz.
The big mistake I think a lot of designers make when implementing full-range drivers is not to equalize the upper rise. If you don’t, it makes the speaker sound thin and lacking bottom end. The trade-off is that the device’s apparent loudness decreases. One way to overcome that issue — especially in some of these small two-driver two-channel Bluetooth devices that have the woofers mounted very close together with no possibility of any stereo phantom center — is to drive them in parallel as a mono source, increasing the product efficiency by 3 dB. If you only drive the two speakers with one amplifier and drive the transducers wired in parallel, you would get a 6-dB increase. The pretense of stereo in some of the tiny Bluetooth speakers seems like bad marketing rather than good engineering.
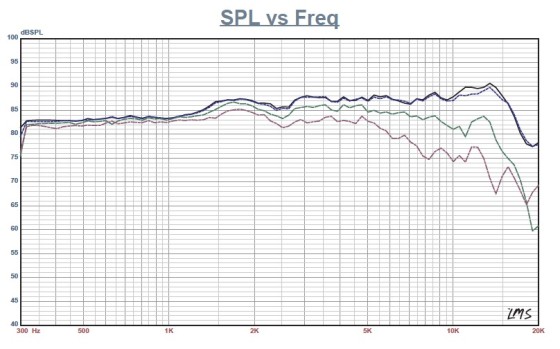
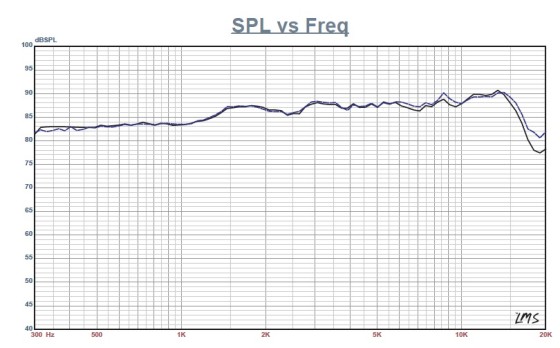
Figure 11 shows the on- and off-axis frequency response at 0°, 15°, 30°, and 45°. The roll-off at 30° off-axis is almost as good as a 1” dome, so I expect the 10F/8414G10’s full-range fidelity to be good. Figure 12 shows the two-sample SPL comparison, which is the 10F/8414G10’s final SPL measurement.
The comparison reveals it is a close match, within less than 1 dB throughout the operating range. For the remaining tests, I employed the Listen SoundCheck AmpConnect analyzer with the Listen 0.25” SCM microphone and power supply to measure distortion and generate time frequency plots. For the distortion measurement, I mounted the 10F/8414G10 rigidly in free air and used a noise stimulus to set the SPL to 94 dB at 1 m (7.2 V). I measured the distortion with the microphone placed 10 cm from the dust cap. Figure 13 shows the distortion curves.
I used SoundCheck to get a 2.83-V/1-m impulse response and imported the data into Listen SoundMap time-frequency software. Figure 14 shows the resulting cumulative spectral decay (CSD) waterfall plot. Figure 15 shows the Wigner-Ville plot, (which I use for its low-frequency performance). While the intended application for this Scan-Speak 3.5” full-range driver is TV, multi-media, and lifestyle speakers, I suggest that, like the Scan-Speak 5F/8422T01, the 10F/8414G10 makes a great driver for line source applications.
www.scan-speak.dk
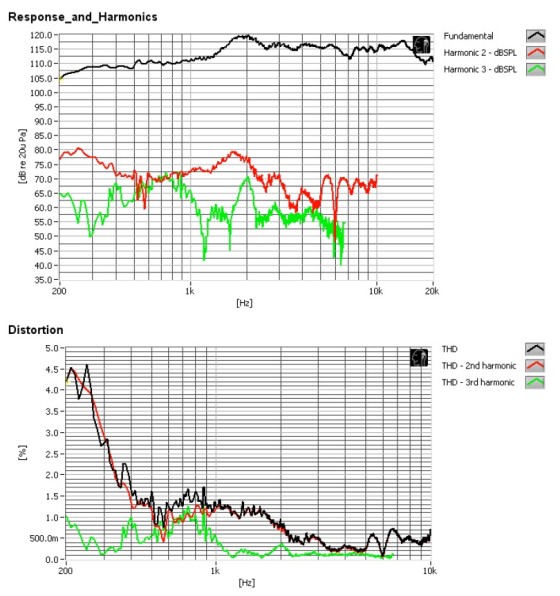
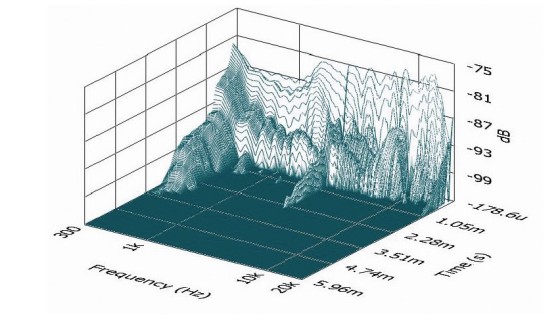
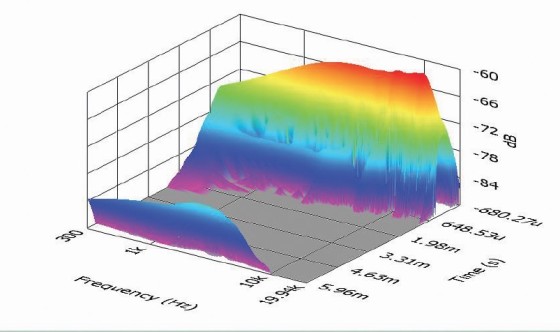